Chapter contents:
Evolution and the Fossil Record
– 1. Natural selection
– 2. Species and species concepts
– 3. Speciation
– 4. Punctuated equilibria and stasis
–– 4.1 Videos about punctuated equilibrium and stasis
– 5. Macroevolution
–– 5.1 Hierarchies
–– 5.2 Species selection
–– 5.3 Abiotic vs. biotic causes of macroevolution
–– 5.4 Evolutionary radiations ←
Patterns of evolutionary radiations
Some clades of organisms are much more species rich than their sister taxa. Such differences in diversity are attributed to rapid evolutionary radiations within the more diverse clades. (Note that usage here of the word radiation has nothing to do with radioactivity! Instead, it means extensive proliferation.) Examples of modern clades that have undergone evolutionary radiations include Hawaiian silversword plants, Hawaiian honeycreeper birds, African cichlid fishes, and Caribbean anole lizards.

Examples of groups that have undergone evolutionary radiations. 1, Mauna Kea Silversword, Argyroxiphium kauense; image by "Naux" (Wikimedia Commons; public domain). 2, 'I'iwi, a species of Hawaiian honeycreeper, Drepanis coccinea; image by the Alan Schmierer, United States Fish and Wildlife Service (Wikimedia Commons; public domain). 3, the East African cichlid fish Tropheuss moorii; image by "Bobby 111" (Wikimedia Commons; Creative Commons Attribution-Share Alike 3.0 Unported license). 4, the Green Anole, Anolis carolinensis; photographer unknown (Wikimedia Commons; Creative Commons Attribution-Share Alike 3.0 Unported license.
A given clade (let's call it Clade A) might appear to be more diverse than its sister taxon (Clade B) for one of two reasons. It could be the case that Clade A has a higher rate of speciation than Clade B. Alternatively, Clade A might have a lower rate of extinction than Clade B, thus resulting in the accumulation of more species over time (because fewer have gone extinct). Only the first possibility (higher rate of speciation) constitutes a true evolutionary radiation. The latter case (lower rate of extinction) has been termed a pseudoradiation by Simões et al. (2016). The processes that drive evolutionary radiations (see below) are very different from those that result in pseudoradiations. How then should one distinguish the two? Fundamentally, it is necessary to demonstrate that an evolutionary radiation has been caused by a high rate of speciation in one clade relative to its sister taxon.
Processes that drive evolutionary radiations
Many different processes can cause groups to undergo dramatic proliferations that result from an elevated speciation rate. One of these is the phenomenon of species selection (see section 5.2 of this chapter). Species selection may result in an evolutionary radiation not because organisms within a given group are more fit (due to natural selection), but instead because the species they belong to are more likely to speciate. Another process that can result in a higher speciation rate has been discussed in the previous section where we described the Turnover Pulse Hypothesis. In Turnover Pulse events, episodes of climate change cause populations of species that belong to multiple groups to become geographically isolated, leading to an increase in rates of speciation. Therefore, Turnover Pulses produce “climatic radiations.”
Adaptive radiation is the most frequently discussed cause of evolutionary radiation in the scientific literature. The increased rate of diversification in adaptive radiations is triggered by biotic factors and primarily involves sympatric speciation (i.e., speciation in geographic proximity). In such cases, species diverge in their morphology and preferred habitat, such that they come to compete less with one another over time. Darwin's finches, which were introduced in the introductory section of this chapter, provide a classic example of an adaptive radiation: the descendants of an ancestral tanager (not, as it turns out, a type of finch) diversified into different ecological niches on the Galapagos Islands in order to reduce competition for limited resources.
Another type of evolutionary radiation frequently encountered in the scientific literature is a geographic radiation. In a geographic radiation, diversification is triggered by the existence of numerous opportunities for geographic isolation that lead to speciation. These are especially apt to occur in regions that are geologically complex, such as tectonically active island chains or mountainous regions, and are thus fueled by increased opportunities for geographic isolation.
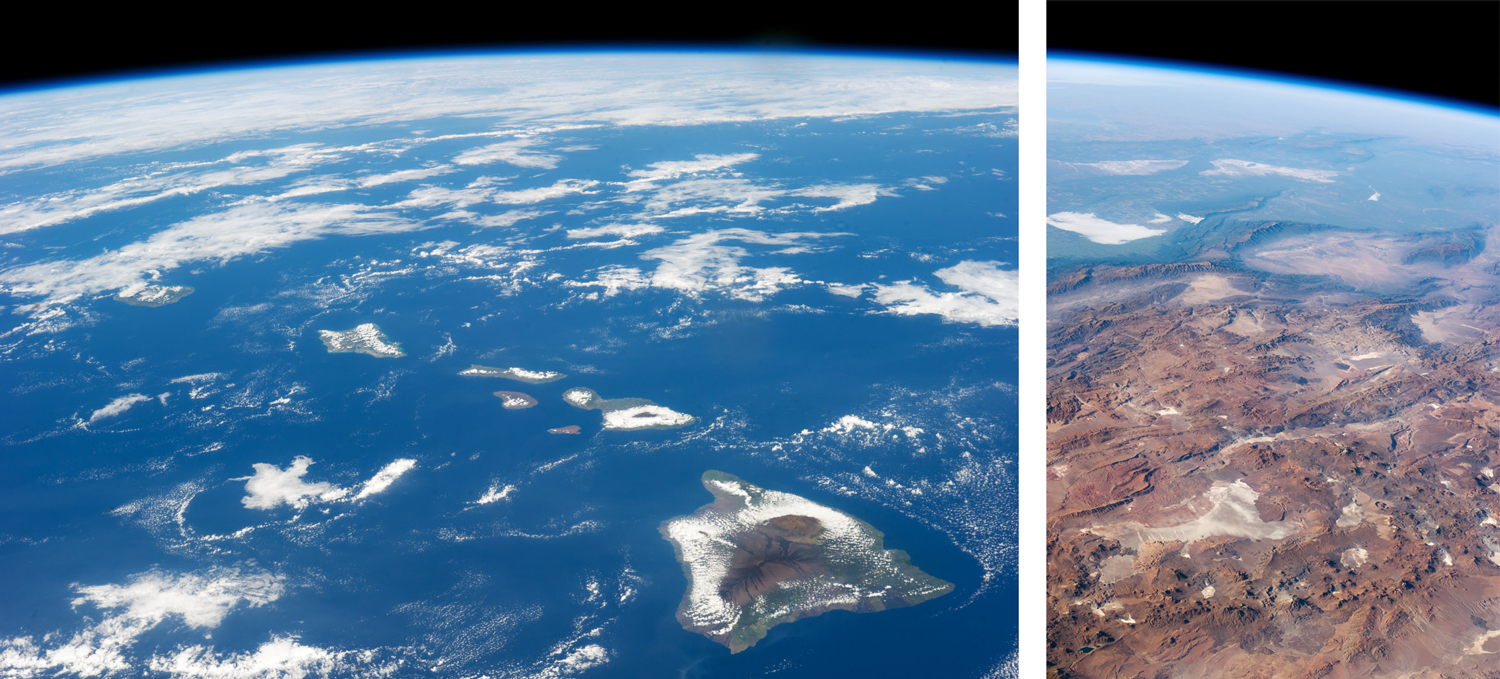
Left, the Hawaiian island chain, photographed by an astronaut aboard the International Space Station (NASA; public domain). Right, the central Andes Mountains, photographed facing eastward (towards Argentina) by an astronaut aboard the International Space Station (NASA; public domain).
The process of geographic isolation may be why island chains often serve as sites of evolutionary radiation. Note that phenomena that might lead to geographic radiation in one type of organism might not necessarily lead to radiation in a different type. This is because certain types of ecologies and dispersal abilities will make populations of species more or less likely to become isolated in general and thus more or less prone to speciate.
One way of thinking about the different processes that drive evolutionary radiations involves distinguishing between the relative role(s) that biotic as opposed to abiotic factors play in causing the increase in speciation rate. For instance, the existence of an adaptive radiation implies a prominent role for biotic factors such as competition and sympatric speciation. By contrast, a geographic radiation implies a prominent role for abiotic factors, such as tectonic forces, that lead to increased opportunities for geographic isolation.
It is important to recognize that both abiotic AND biotic factors are often involved in evolutionary radiations. This is because several different processes may interact to produce the radiation. A likely example involves African cichlid fishes (see image at the top of this page). These fishes occur in geographically complex lakes in a geologically dynamic rift valley that creates numerous opportunities for the isolation of aquatic species.
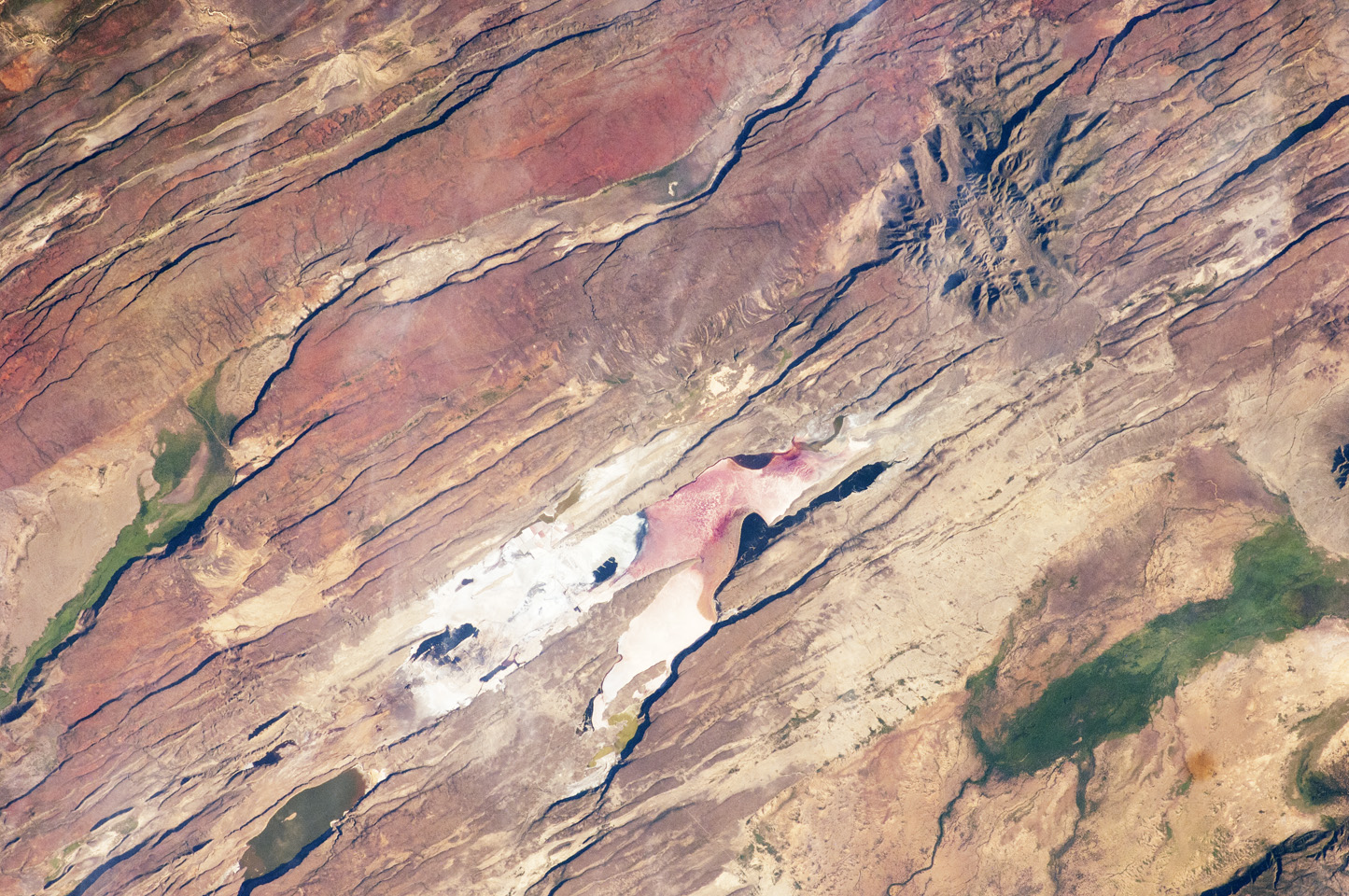
The East African Rift Valley; some low-lying areas within the rift are filled with water, forming freshwater lakes (the bright-white region is sunlight reflecting off of Lake Magadi. Photograph captured by an astronaut aboard the International Space Station (NASA; public domain).
One process fueling this evolutionary radiation is geographic in nature and is ultimately caused by abiotic tectonic forces that create basins that can fill with water, forming lakes. Furthermore, climate in this region has cycliced repeatedly between wet and dry conditions. During drier times, larger lakes would fragment into many small, isolated lakes, which would become cauldrons for geographic isolation and speciation. During wetter periods, these lakes reconnected and the newly diverged species expanded their ranges into the larger, continuous lakes. The cycle would then repeat as the climate dried again. Thus, African cichlids might also comprise a climatic radiation.
That might not, however, be the end of the story. There is also likely a biotic factor that makes cichlids more likely to speciate. Cichlids have complex behaviors associated with their breeding systems that might favor the formation of reproductive groups that are apt to evolve rapidly. Thus, the African cichlid radiation may also involve an example of species selection. This is supported by the fact that there are other groups of fishes in the region that are far less diverse than the cichlids, despite living in a similar environmental setting.
We see from this example that complex factors may interact in many different ways to produce an evolutionary radiation. This is one of the exciting aspects of evolutionary radiations, and likely one of the reasons why so many scientists are interested in studying them. Scientific study of evolutionary radiations is not, of course, restricted to the living biota. Paleontologists have uncovered many episodes of dramatic diversification across the history of life, from the Cambrian radiation of animals to the diversification of mammals following the end-Cretaceous mass extinction. Intriguingly, the phenomenon of adaptive radiation was first introduced by the famous paleontologist Henry Fairfield Osborn to explain the proliferation of certain Cenozoic mammal groups. And some of the most notable instances of diversification in the history of life can be seen in the evolutionary radiations of fossil groups like Cambrian trilobites and Mesozoic ammonoids.
Left, the Cambrian trilobite Wanneria walcottana from the Kinzers Formation of Lancaster County, Pennsylvania. Right, the Cretaceous ammonite Discoscaphites conradi from the Fox Hills Formation of South Dakota. Scale bars equal 1 cm. Both specimens are from the collections of the Paleontological Research Institution, Ithaca, New York. Image by Jonathan R. Hendricks.
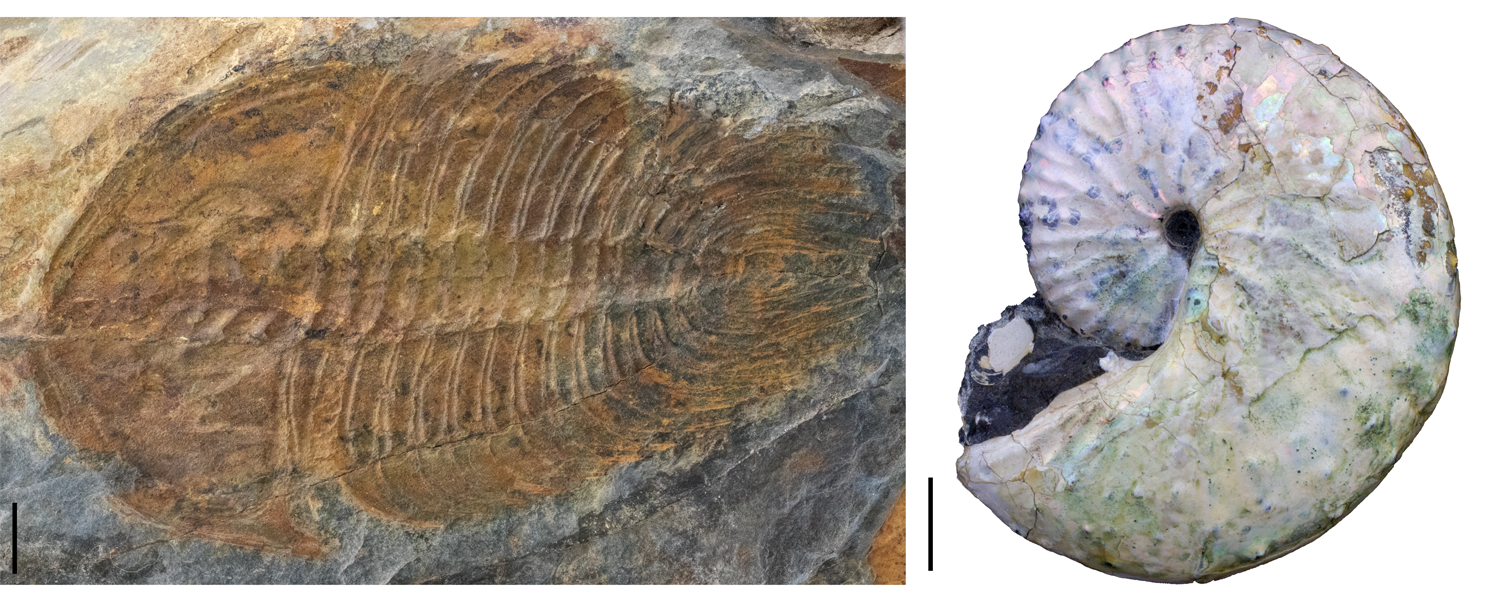
Equally staggering is that each of these tremendously species-rich groups are now entirely extinct. This matches a more universal pattern also common to human existence: sometimes you’re up and sometimes you're down; sometimes you’re the pigeon, other times the statue; every dog has its day, and some days are longer than others. There are two key sides of the coin when it comes to understanding the evolutionary histories of major clades of organisms: why, and how much, are they speciating?; and why, and how fast, are they going extinct? It is this delicate balance and complex interplay that determines a clade’s fate, be it long-term burgeoning or prolonged withering. This is why research on both fossil and extant organisms will continue to produce fundamental insights into this key area of macroevolution.
References and further reading
Eldredge, N., and J. Cracraft. 1980. Phylogenetic Patterns and the Evolutionary Process. Columbia University Press, New York, NY.
Lieberman, B. S. 2012. Adaptive radiations in the context of macroevolutionary theory: a paleontological perspective. Evolutionary Biology 39:181-191.
Losos, J. B. 2009. Lizards in an Evolutionary Tree: Ecology and Adaptive Radiation of Anoles. University of California Press, Berkeley, CA.
Simões, M., Alvarado, M., Breitkreuz, L, Baca, S., Cooper, J. C., Heins, L., Herzog, K., and B. S. Lieberman. 2016. The evolving theory of evolutionary radiations. Trends in Ecology & Evolution (TREE) 31:27-34.
Content usage
Usage of text and images created for DEAL: Text on this page was written by Bruce S. Lieberman and Jonathan R. Hendricks. Original written content created by Bruce S. Lieberman and Jonathan R. Hendricks for the Digital Encyclopedia of Ancient Life that appears on this page is licensed under a Creative Commons Attribution-NonCommercial-ShareAlike 4.0 International License. Original images created by Jonathan R. Hendricks are also licensed under Creative Commons Attribution-NonCommercial-ShareAlike 4.0 International License.
Content sourced from other websites: Attribution, source webpage, and licensing information or terms of use are indicated for images sourced from other websites in the figure caption below the relevant image. See original sources for further details. Attribution and source webpage are indicated for embedded videos. See original sources for terms of use. Reproduction of an image or video on this page does not imply endorsement by the author, creator, source website, publisher, and/or copyright holder.