Chapter by:
Jonathan R. Hendricks, Paleontological Research Institution, Ithaca, New York
This chapter was lasted updated by the author on May 22, 2019.
Chapter citation:
Hendricks, J. R. 2019. Animal phylogeny. In: the Digital Encyclopedia of Ancient Life. https://www.digitalatlasofancientlife.org/learn/animal-phylogeny/
Documenting the broad evolutionary history of animal, or Metazoan, life has been a central goal of biology and paleontology since the early work of Lamarck (1744–1829) and Ernst Haeckel (1834–1919). Numerous competing hypotheses of relationships amongst the 30-some recognized animal phyla have been developed over the past two centuries, most based on the anatomical and developmental characteristics of their constituent animal species.
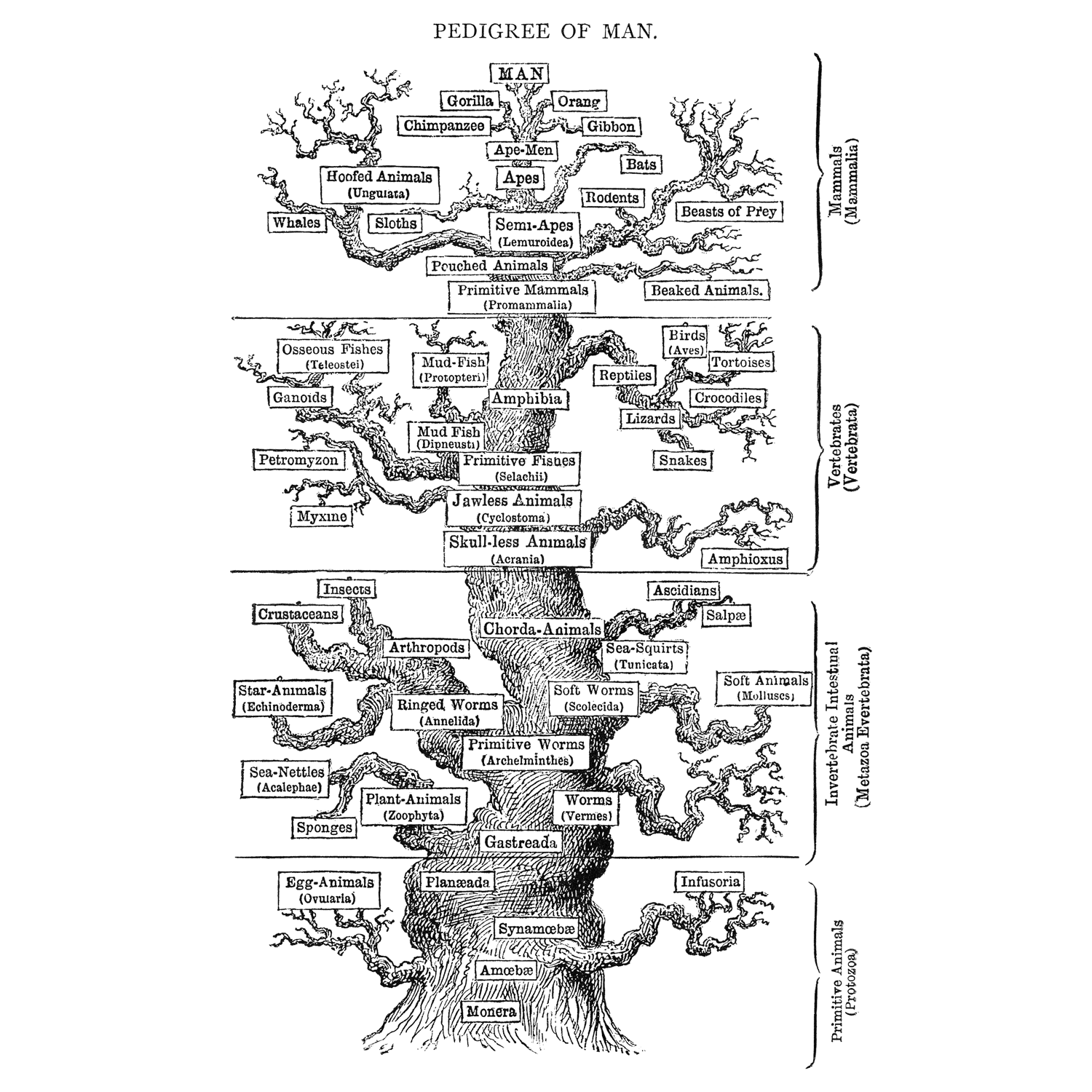
Ernst Haeckel's hypothesis for animal phylogeny, published in 1879 (Wikimedia Commons; public domain). This phylogenetic hypothesis retains little in common with modern hypotheses of animal relationships. Note also the construction of the tree, which places vertebrates, and especially humans, at the top, implying their superiority over other types of animal life. We now recognize chordates as just one of many diverse branches in the animal tree of life. They are not superior in any particular way, but rather bear their own unique combinations of synapomorphies that differentiate them from other groups.
The advent of molecular phylogenetic systematics—especially from the late 1980's through the late 1990's—began to provide some clarification regarding the major features of Metazoan phylogeny. Some long-held hypotheses of relationships were supported by genetic data, while others were found to have little support at all. See the reference list below for research papers that were especially significant during this period, as well as several useful broad reviews of animal phylogeny. (Telford et al., 2015 provides an especially helpful recent overview of animal phylogeny, its history of discovery, and outstanding questions).
The following chapters of this textbook cover only paleontologically-significant animal phyla and these chapters are arranged phylogenetically. This page, therefore, is meant to serve as a highly simplified introduction to the major features of animal evolution as suggested by recent molecular phylogenetic research, as well as the relationships amongst those groups with significant fossil records. It is recommended that you have familiarized yourself with phylogenetic terminology and how cladograms work before exploring this chapter (Systematics chapter here).
Major features of animal phylogeny
The phylogenetic framework followed here is derived and highly simplified from the molecular phylogenetic hypothesis recently presented by Telford et al. (2015). Importantly, the phylogenetic tree below omits numerous animal phyla that left behind little or no fossil record. (Most of these animal phyla are small bodied and lack hard parts, making their likelihood of being fossilized, much less discovered, exceedingly unlikely.)
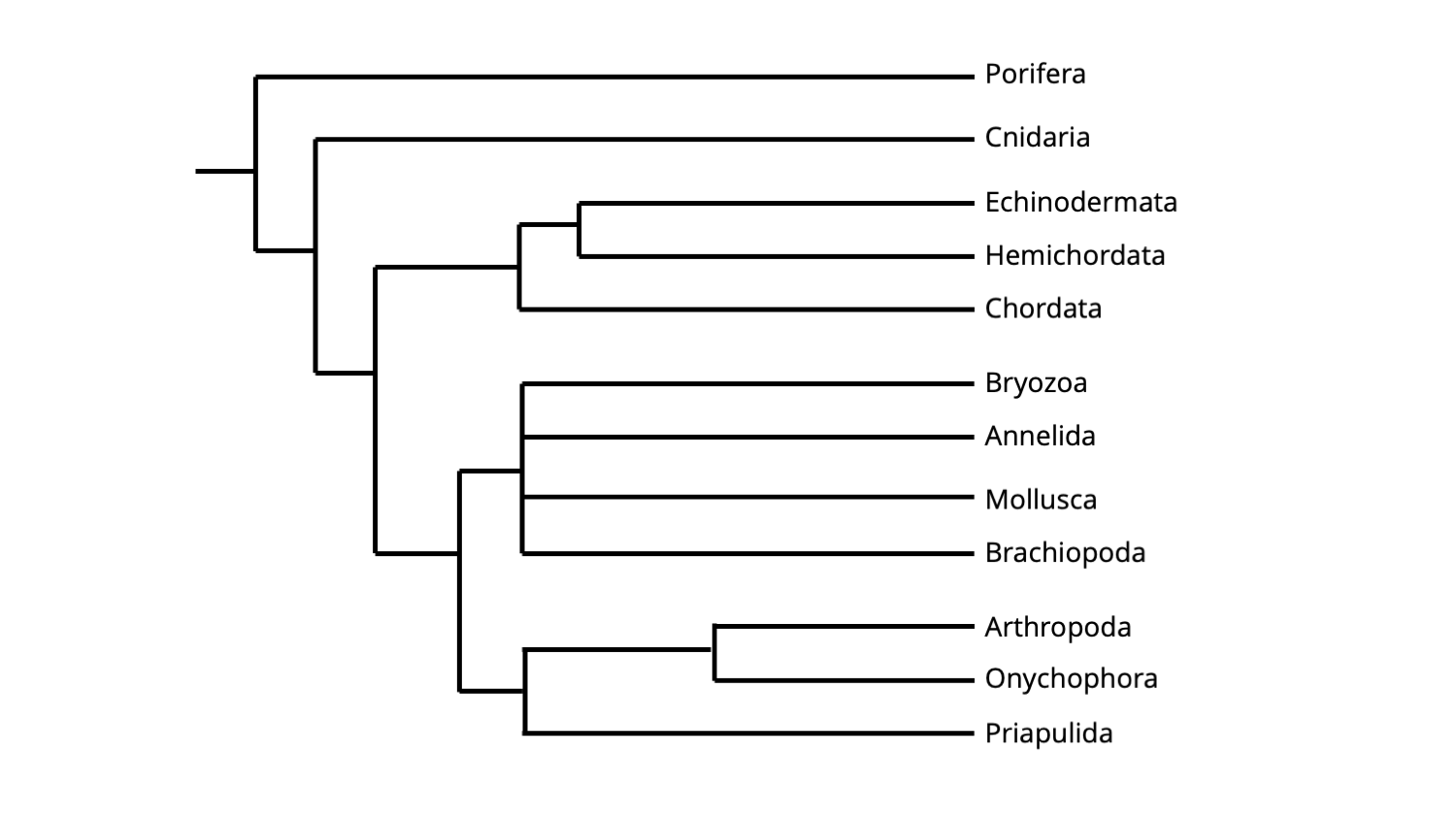
Major framework of animal phylogeny, highly simplified from version published by Telford et al. (2015). Image by Jonathan R. Hendricks.
Before considering the major clades of animal life, let's introduce some examples of the paleontologically-significant phyla that form the "tips" of this phylogenetic tree:
- Porifera: sponges.
- Cnidaria: jellyfish, corals, sea anemones, Hydra, and others.
- Echinodermata: starfish, sea urchins, crinoids, and others.
- Hemichordates: a somewhat obscure group that includes the paleontologically-signficant graptolites.
- Chordata: you, your dog, your cat, your goldfish, your lizard, your parakeet, the squirrel outside, etc. (animals with backbones, plus a few others).
- Bryozoa: moss animals; small, colonial animals that are paleontologically significant.
- Annelida: many groups of "worms," including earthworms and many marine forms.
- Mollusca: snails, squids, ammonites, octopuses, bivalves, etc.
- Brachiopoda: the "lamp shells," which are obscure today, but once had a very important fossil record.
- Arthropoda: insects, spiders, crabs, copepods, centipedes, etc.
- Onychophora: velvet worms; a group with a small, but interesting fossil record.
- Priapulida: penis worms (seriously); a small group of predatory worms that is important for understanding arthropod evolution.
The major groupings, or clades, that comprise animal phyla are very briefly introduced below. Additional details about individual phyla are covered in later chapters.
Porifera
In most analyses, phylum Porifera (sponges) forms the base of the animal tree of life, meaning that sponges are the sister group of all other animal life.
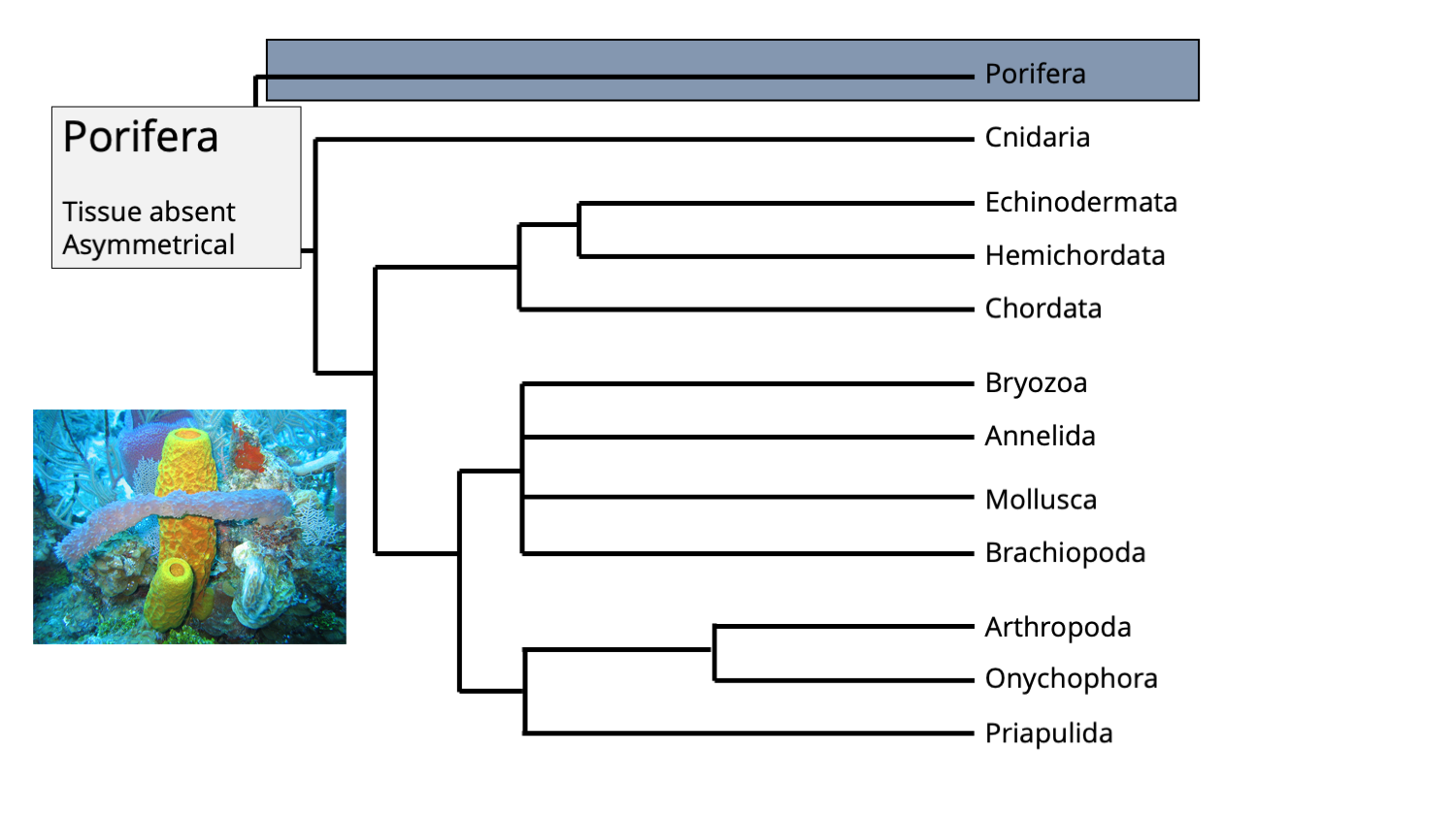
The position of phylum Porifera in the animal tree of life. Phylogenetic tree Jonathan R. Hendricks; inset image of modern sponges by NOAA (Creative Commons; public domain).
Sponges are very different from most other groups of animals, most particularly because they 1) lack true tissue and 2) are asymmetrical in shape. They also lack a nervous system and do not have any opening that could be characterized as a mouth. In many respects, they can be thought of as a collections of quasi-independent cells that form a semi-cohesive whole.
Eumetazoa
The Eumetazoa clade represents the broadest grouping within the animal evolutionary tree. It includes all animals except Porifera. All eumetazoans have true tissues, which are grouping of similar cells that carry out specialized functions in the body. These are organized into germ layers early in development, which give rise to other tissues and organs. Additionally, eumetazoans have neurons (i.e., a nervous system).
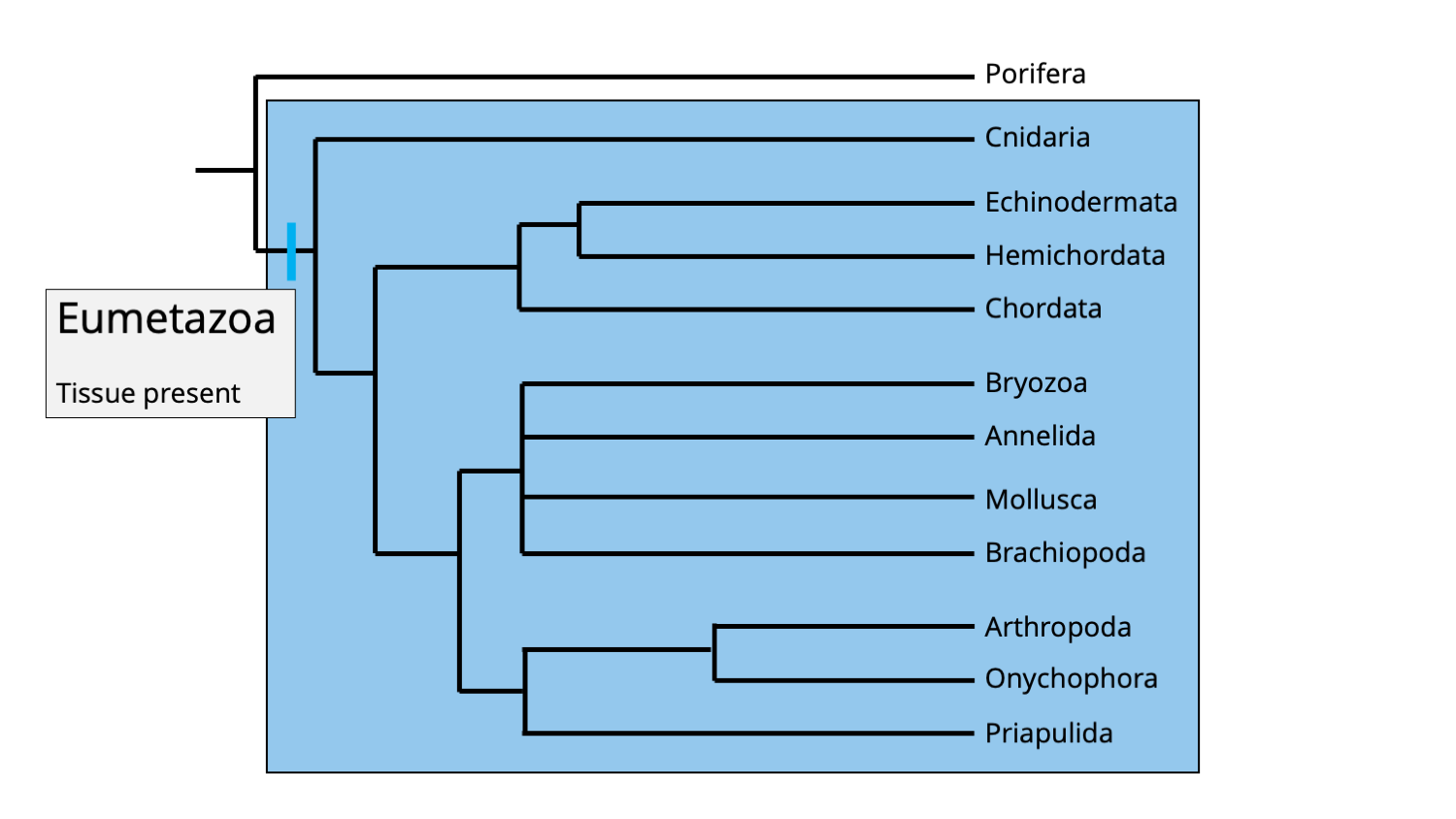
The Eumetazoa clade includes all animals, except phylum Porifera. A feature shared in common by all eumetazoans is the presence of true tissues. Image by Jonathan R. Hendricks.
Cnidaria
Cnidaria represents the basal-most clade of eumetazoans, and includes jellyfish, sea anemones, corals, and other forms.
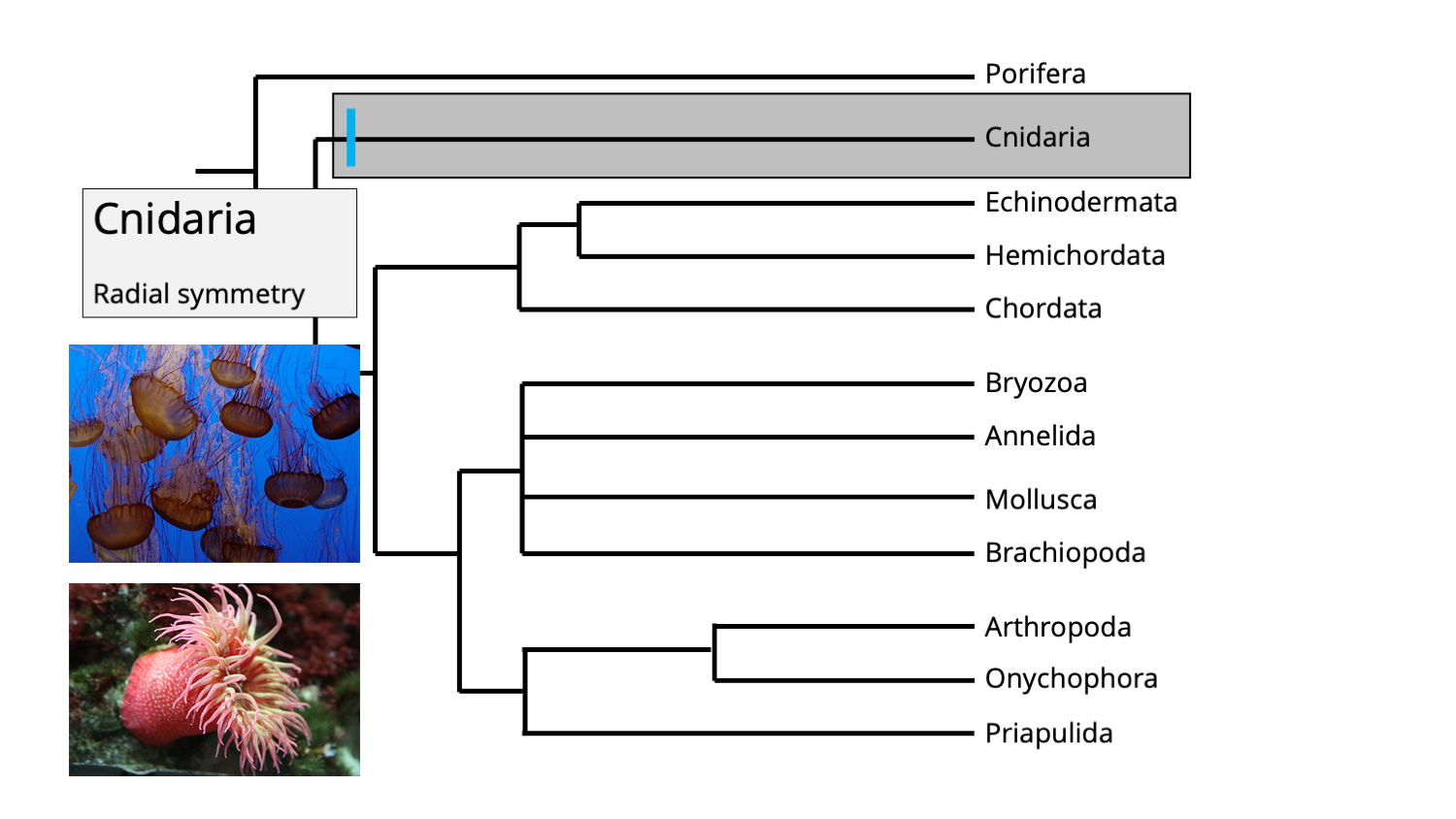
The position of phylum Cnidaria amongst the animal tree of life. Inset images show jellyfishes (top) and a sea anemone (bottom), both of which are cnidarians. Image by Jonathan R. Hendricks.
One synapomorphy for the cnidarian clade is the presence of radial symmetry, which separates them from all other eumetazoans (which have bilateral symmetry; see below). Consider the illustration of the sea anemone (a type of cnidarian) below. Imagine cutting it like a birthday cake (gross!): no matter how you slice it through the middle, one half is the mirror image of the other.
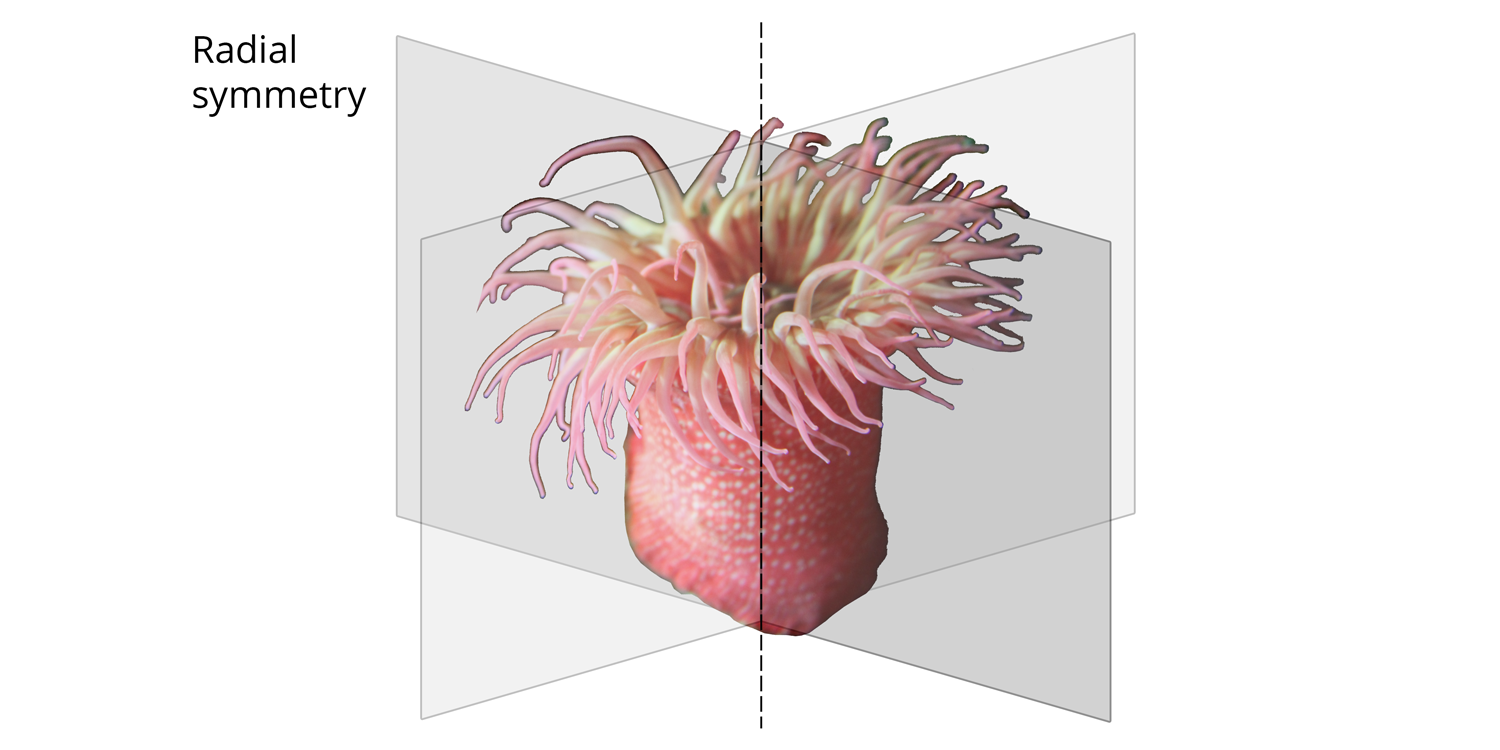
Illustration of radial body symmetry in a sea anemone. Each plane of symmetry divides the sea anemone into a half that is the mirror image of the other half. All cnidarians have this type of symmetry. Image by Jonathan R. Hendricks.
There are additional synapomorphies of cnidarians that are covered in the corresponding chapter of this textbook.
Bilateria
Bilaterians include all eumetazoans, except for cnidarians.
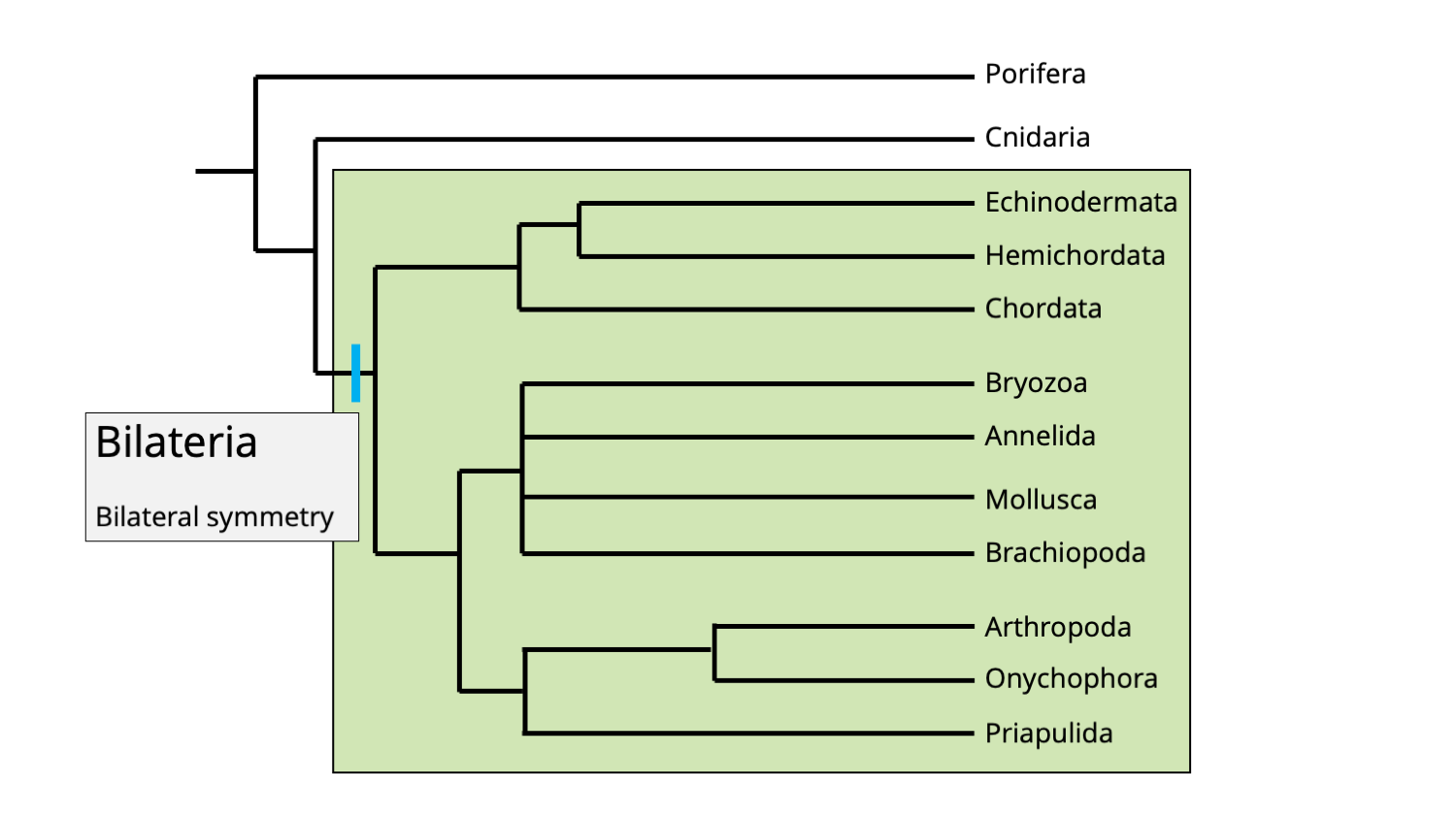
The Bilateria clade includes all eumetazoans, except phylum Cnidaria. A feature shared in common by all bilaterians is bilateral symmetry. Image by Jonathan R. Hendricks.
The unifying synapomorphy for bilaterians is easy to remember: their bodies have bilateral symmetry. This means that there is only one plane of symmetry that will make one side the mirror image of the other. (Note that this is somewhat of a generalization; humans are more-or-less bilaterally symmetrical on the outside, but many of our internal organs—such as the heart and liver—are asymmetrical in both shape and position.)
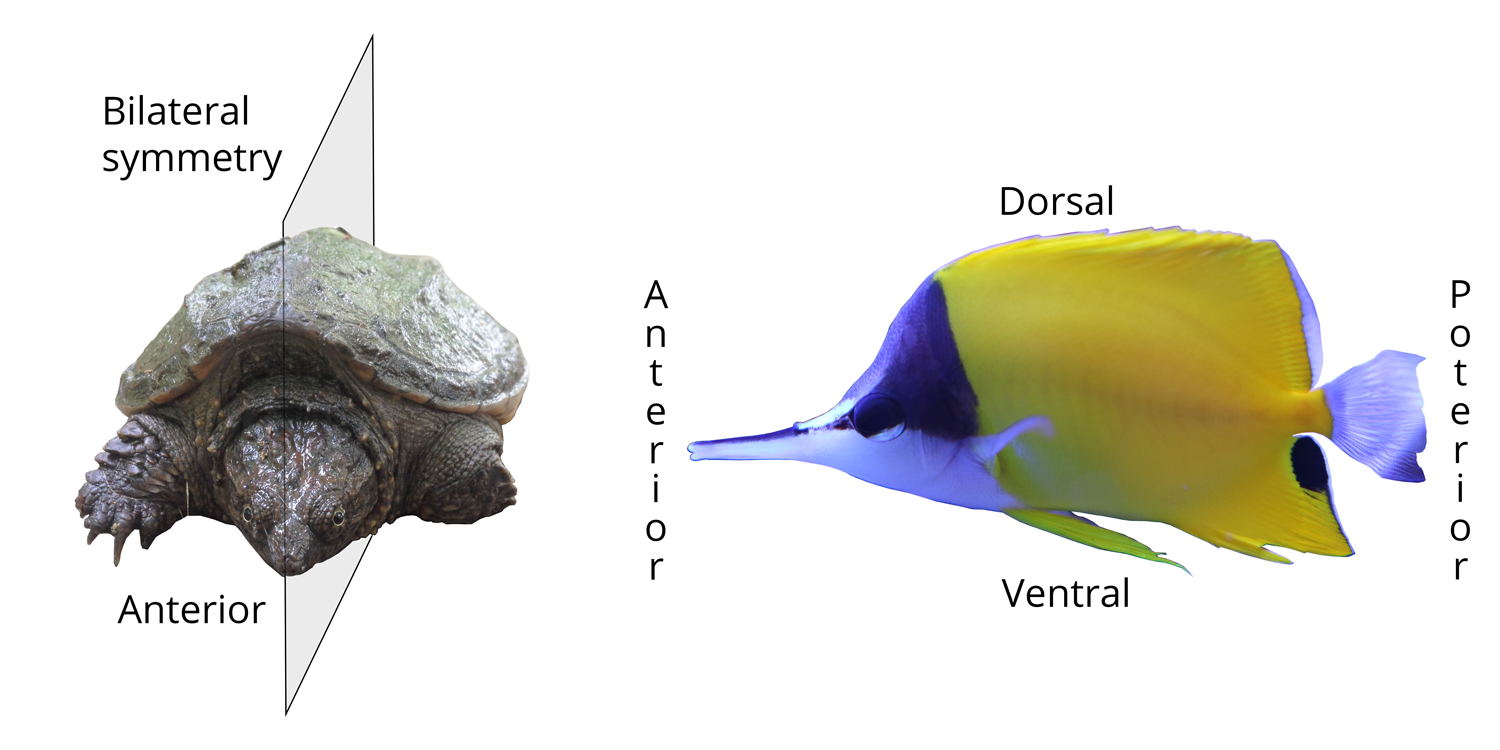
Left: illustration of bilateral body symmetry in a juvenile snapping turtle; the plane of symmetry makes one side the mirror image of the other. Right: positional terms for bilaterally symmetrical animals. The head end is anterior, the tail end is posterior, the back side is dorsal, and the belly side is ventral. Image by Jonathan R. Hendricks.
Unlike cnidarians and sponges, bilaterians also have a head and this is called, by convention, the anterior end of the animal. The opposite end, the "butt end," is posterior. The top side of bilaterians is dorsal (think of the dorsal fin of a shark, which sticks out of the water) and the belly side is ventral.
Protostomia and Deuterostomia
Bilaterians are divided into two major clades: Protostomia and Deuterostomia.
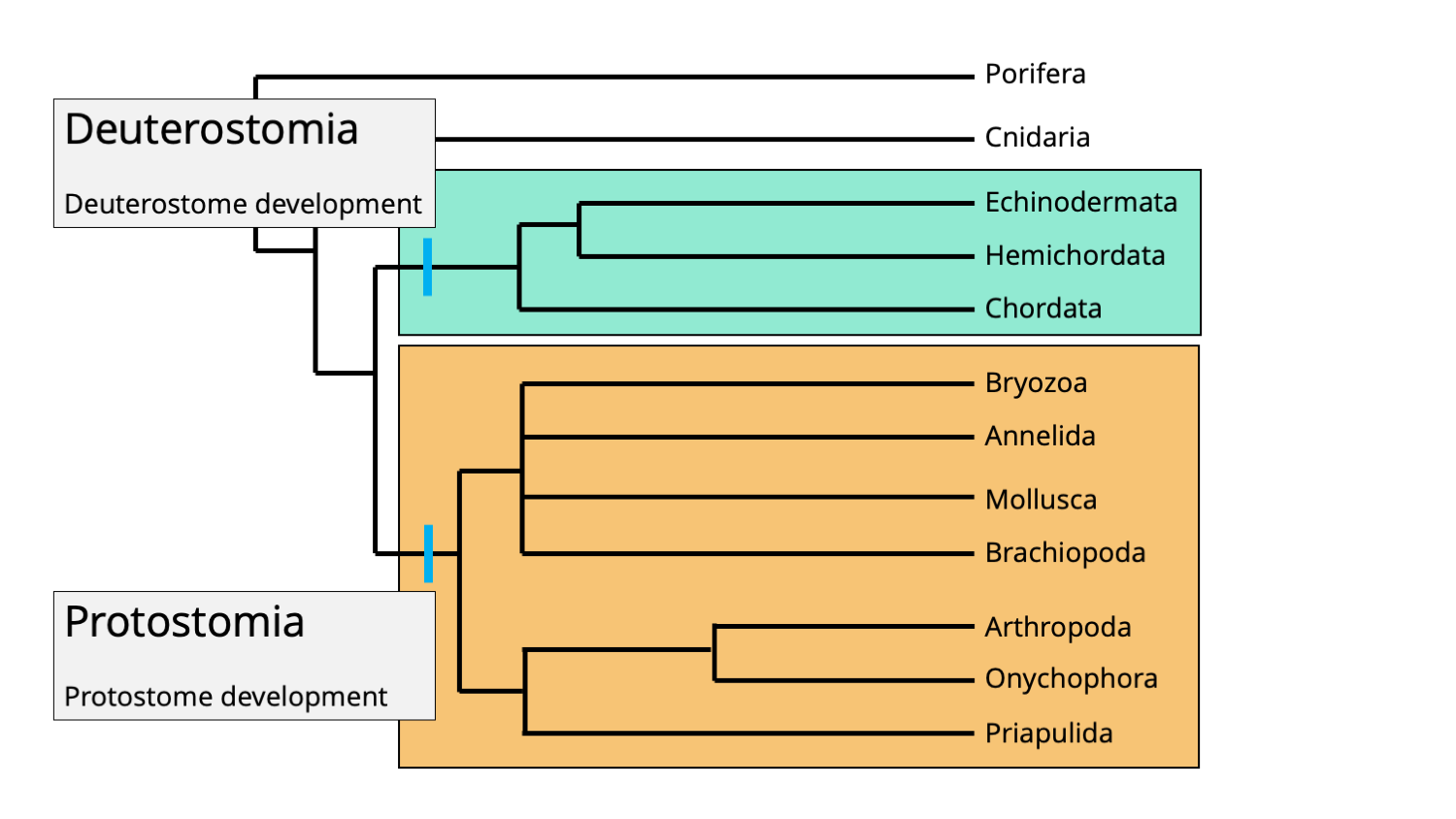
Phylogenetic positions of the bilaterian clades Protostomia and Deuterostomia. Each clade is characterized by its ordering of embryonic development. The mouth forms first in protostomes, but second (after the anus) in deuterostomes. Image by Jonathan R. Hendricks.
Think about the digestive system of most bilaterians as a long tube. At one end of the tube is the mouth; at the other end is the anus. Early in the embryonic development of all bilaterians, an indentation forms that is called the blastopore. In protostomes ("proto" = first; "stome" = mouth), the blastopore becomes the mouth (i.e., "mouth-first"). In deuterostomes ("deutero" = second; "stome" = mouth), the blastopore becomes the anus ("mouth-second").
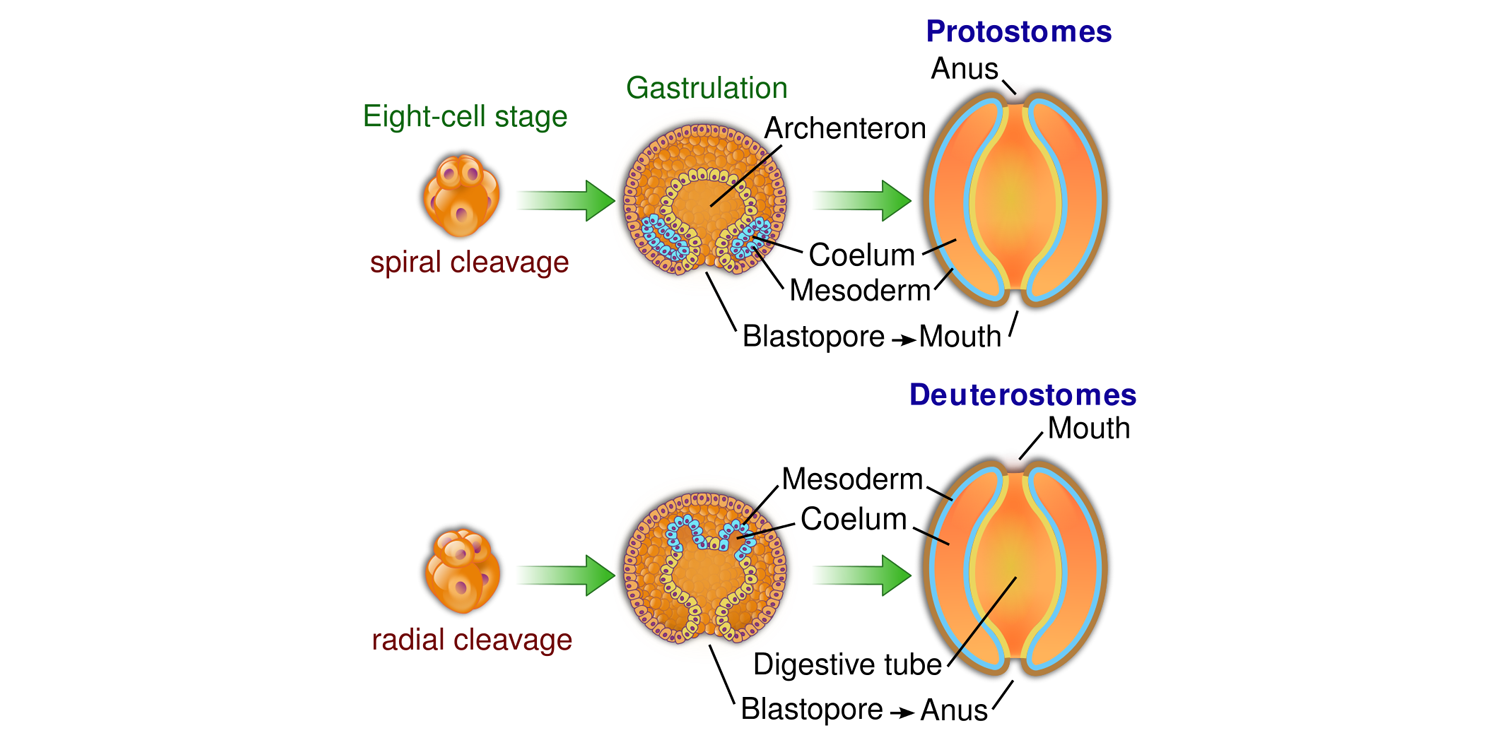
Differing fates of the blastopore in protostomes and deuterostomes. Image by "YassineMrabet" (Wikimedia Commons; Creative Commons Attribution-Share Alike 3.0 Unported license).
Source: "Animal Development: We're Just Tubes - Crash Course Biology #16" (YouTube).
As chordates, humans belong to the deuterostome clade. This means that very early in our development, our anus developed before our mouth. Chordates share this mode of deuterostome development with two other closely related phyla, Hemichordata and Echinodermata. All other bilaterians are protostomes, which are further divided into two clades: Lophotrochozoa and Ecdysozoa.
Lophotrochozoa
Lophotrochozoans are one of the two major clades of protostomes (the other clade is Ecdysozoa). While the clade includes many animal phyla, the four most paleontologically significant are mollusks (e.g., snails, clams, squids, ammonites, etc.), brachiopods (lamp shells), bryozoans (moss animals), and annelids (many types of worms).
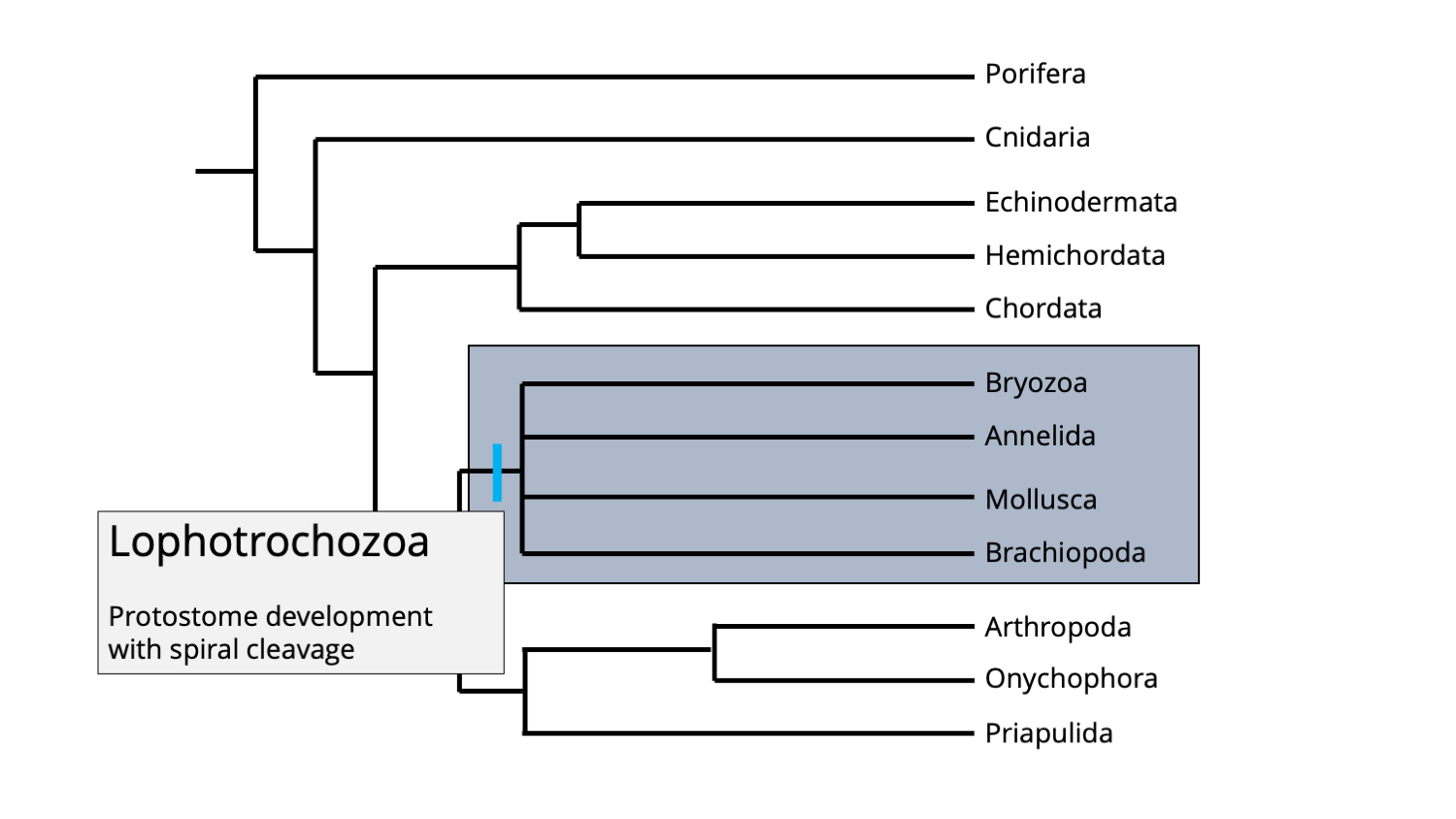
Phylogenetic position of the Lophotrochozoan clade. Image by Jonathan R. Hendricks.
The name of this clade is derived from the fact that its members have either a feeding structure called a lophophore (brachiopods and bryozoans), or have a specialized type of larval form called a trochophore (mollusks and annelids). More generally, members of the Lophotrochozoa all have a special style of cell cleavage early in their development. Cleavage concerns how the fertilized egg cell (the zygote) divides into more and more cells, which are called blastomeres (first two blastomeres, then four, then eight, and so on). All animals other than lophotrochozoans have radial cleavage. In radial cleavage:
"[T]he mitotic spindles of each successive division are at right angles to those of the preceding division. Usually the first two cleavages are vertical, the third horizontal, so that at the eight-cell stage each blastomere of the upper tier lies directly above one of the blastomeres of the lower tier" (Kozloff, 1990, p. 849). Note this in image E in the image immediate below.

Radial cleavage in the coral Lophelia pertusa. In particular, observe (E), the eight-cell stage. Image is from Larsson et al., (2014) in PLoS ONE (Creative Commons Attribution license). Original caption "Compound microscope images of the embryo development of Lophelia pertusa. (A) oocyte; (B) 1st cleavage; (C) 2-cell; (D) 4-cell; (E) 8-cell; (F) 16-cell morula; (G) 32-cell morula; (H) 64-cell blastula. First cleavage is equal and holoblastic, followed by radial cleavage. A slight shift of blastomeres sometimes made cleavage appear pseudo-spiral. Scale bar 100 µm."
The style of cleavage in lophotrochozoans, called spiral cleavage, is different and somewhat more complicated than that of radial cleavage:
"[A]fter the four-cell stage has been rached, the mitotic spindles are oblique with respect to those of the first two divisions. Thus at the eight-cell stage, the cells of the upper tier are not directly above the cells of the lower tier, but on the furrows between them (Kozloff, 1990, p. 850). Note this in part B (top and bottom) of the image immediately below.
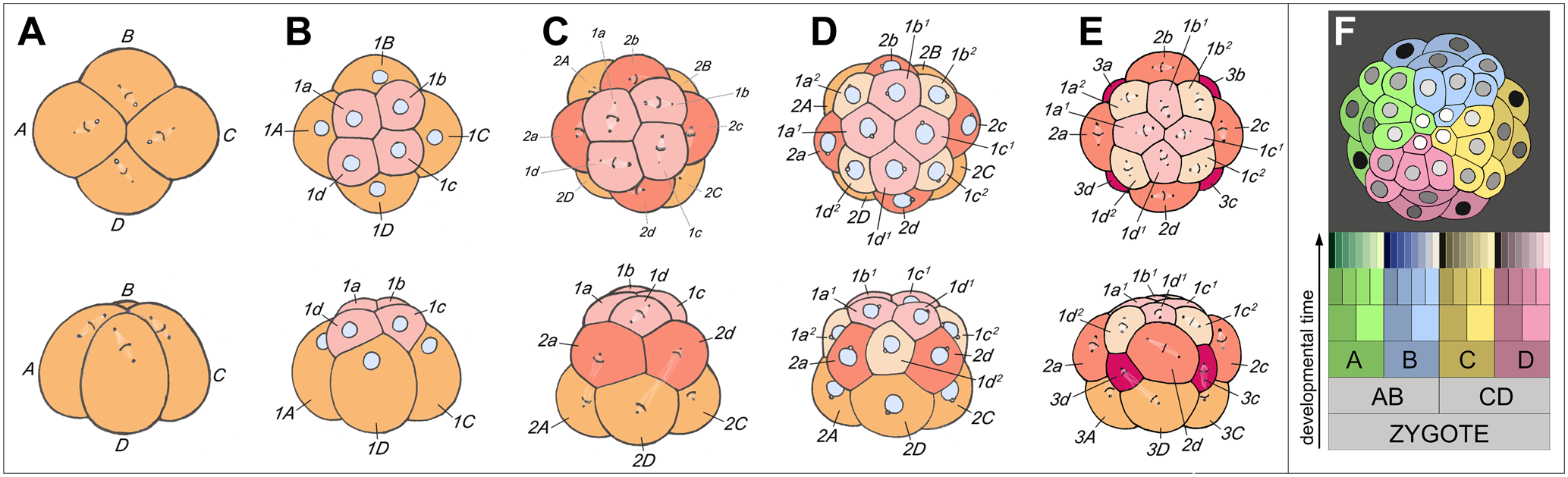
Illustration of spiral cleavage in the snail Trochus. In particular, observe B (top and bottom), the eight-cell stage. Image is from Goulding (2009) in PLoS ONE (Creative Commons Attribution license). Original figure caption: "Spiral cleavage: elaborating fourfold rotational symmetry about the primary axis of polarity. (A) through (E) show the third through fifth rounds of cleavage in the basal gastropod Trochus, as seen from the animal pole (top row) and from one side (bottom row). (A) Mitotic spindles in the quadrant founder cells are skewed clockwise, preparatory to dexiotropic division. (B) Eight-cell interphase; the first-quartet micromeres (1a–1d) are nestled between neighboring macromeres (1A–1D). (C) Formation of the second micromere quartet by laeotropic division; the first-quartet cells in this species also divide laeotropically, but with a slight delay (a much longer delay occurs in Ilyanassa). (D) Sixteen-cell stage: interphase cells are nestled with new neighbors. (D) Fifth cleavage: the third-quartet micromeres are formed by dexiotropic division of macromeres; cells of both the first and second micromere quartets divide also dexiotropically, but with a slight delay. (F) The distribution of quadrants at a later cleavage stage of this relatively simple spiralian embryo. In this animal pole view, the four quadrant lineages are color-coded. First-quartet clones are shaded light, and second-quartet clones dark (After Robert, 1902). The schematic cell lineage table at bottom shows the typical relationships of the quadrants, and indicates the parallel generation of micromeres and micromere sublineages in the four quadrants of a generalized spiralian embryo."
Ecdysozoa
Ecdysozoans represent the other major clade of protostomes, and include arthropods, onychophorans (velvet worms), and priapulid worms. While onychophorans and priapulids are relatively obscure groups, arthropods are the most diverse phylum of animal life and have been important since the Cambrian period.
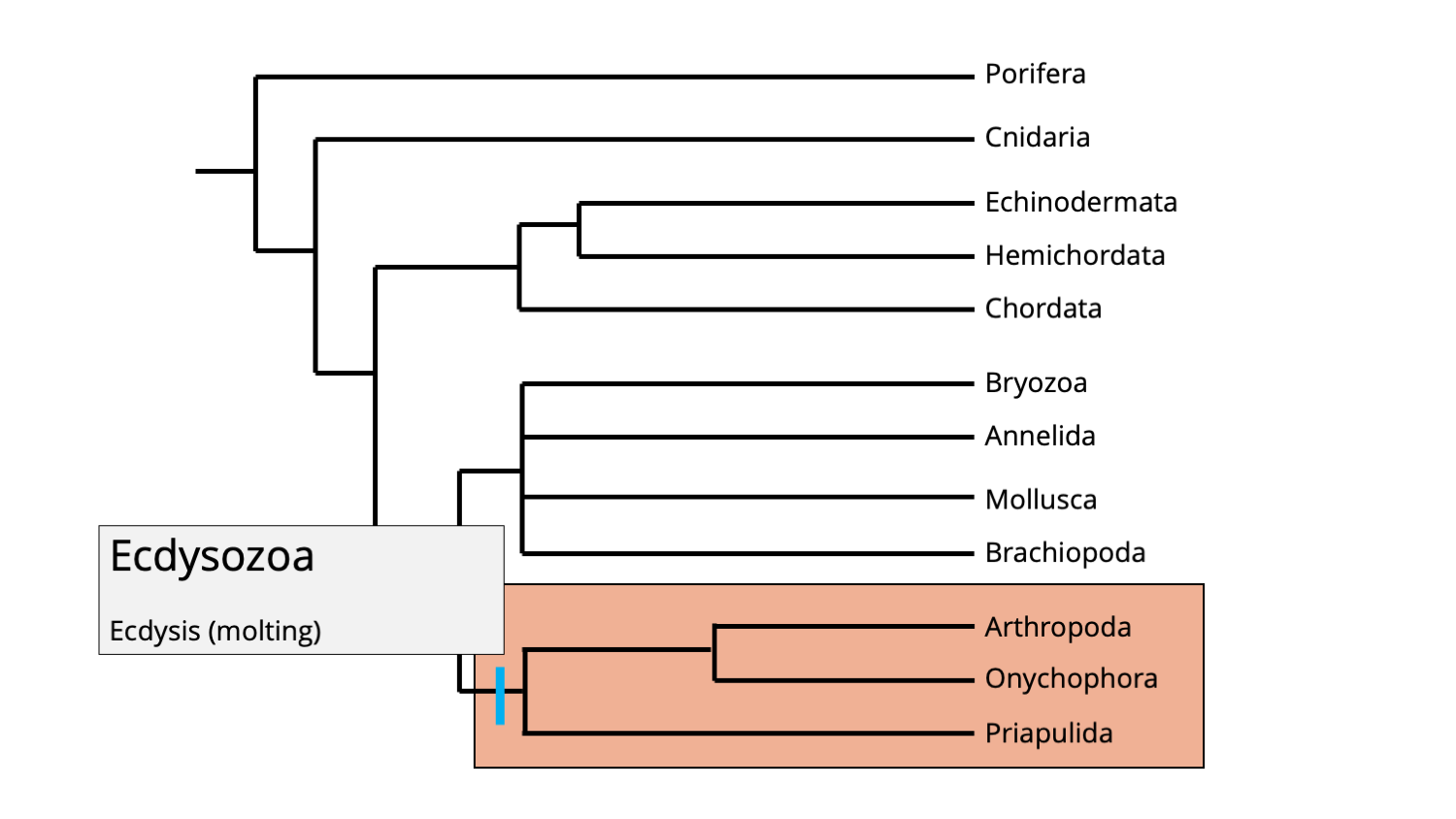
Phylogenetic position of the ecdysozoan clade. Image by Jonathan R. Hendricks.
Members of the ecdysozoa clade are united by the fact that they undergo ecdysis during development, meaning that they molt their exoskeletons as they grow. Molting is a necessity for animals that have hardened or semi-hardened external skeletons because that exterior becomes a barrier to growing larger as the animal ages. (Imagine growing larger within a suit of armor that is too small for you. Over time, it would get quite uncomfortable!) It is not a concern, however, for animals that have internal skeletons (like we do), or animals that do not have any skeletons at all.
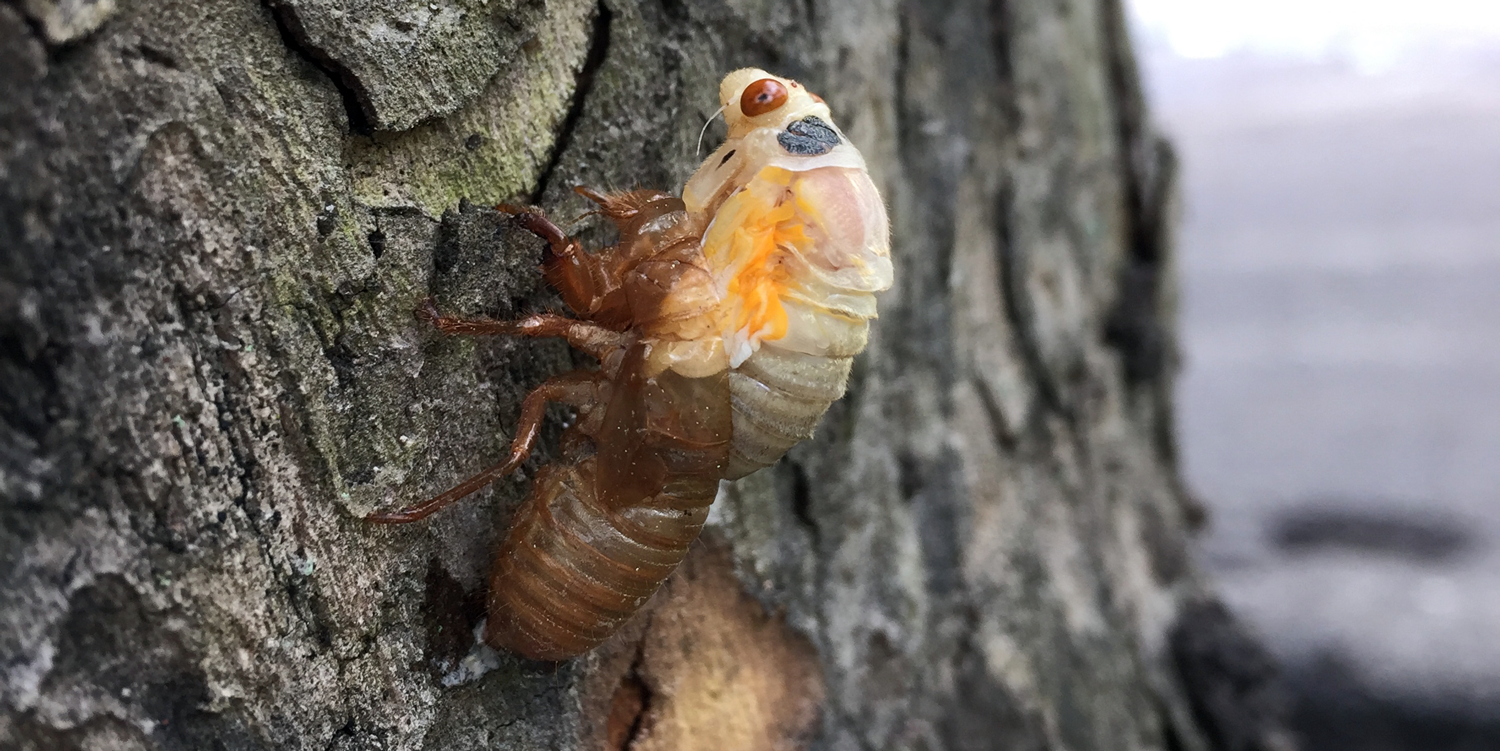
A cicada emerging from its molted exoskeleton. Photograph by Jonathan R. Hendricks.
Summary
The cladogram below shows how the major clades of animals presented above are positioned relative to one another within the broader animal tree of life.
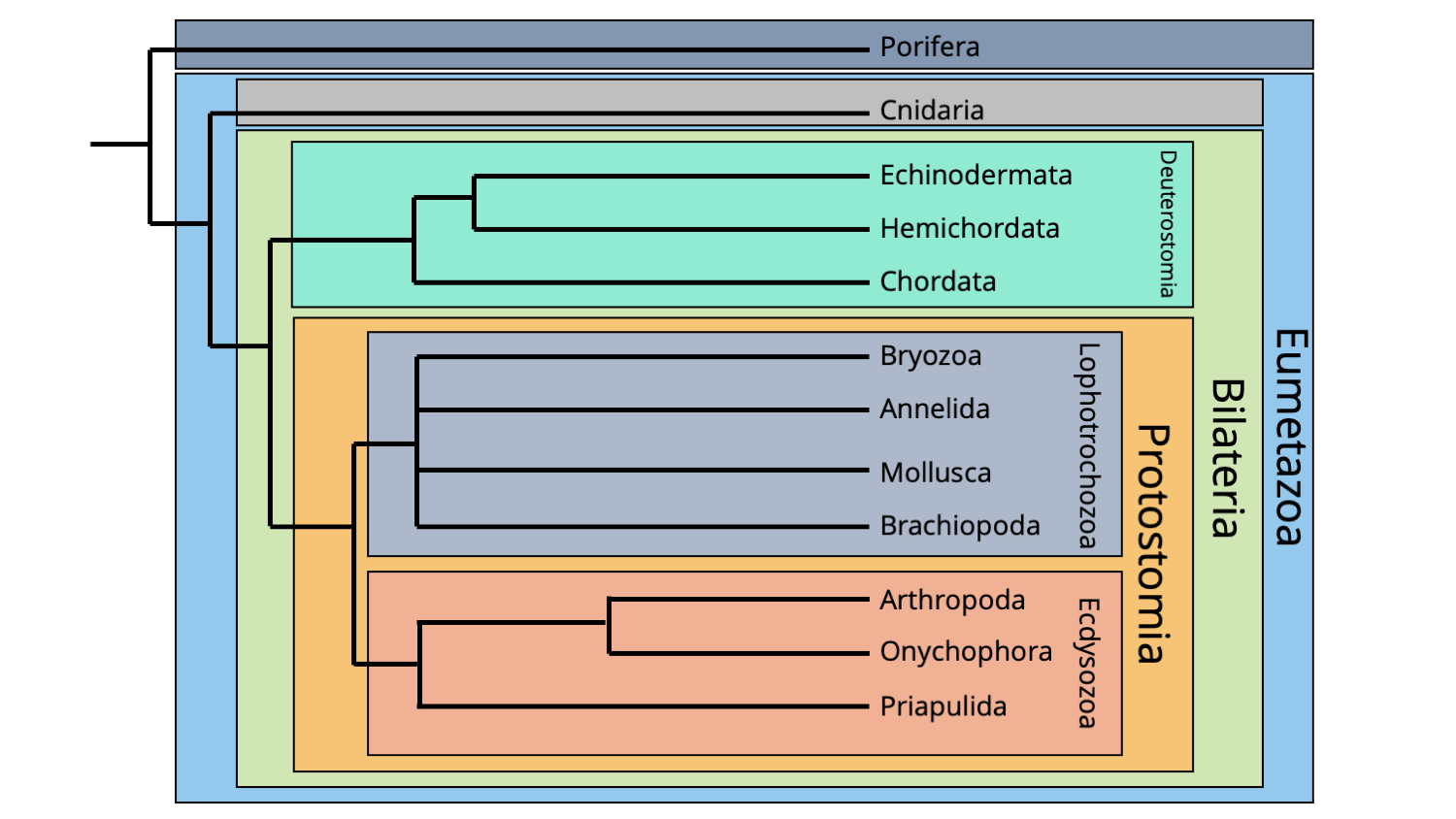
Simplified hypothesis of animal phylogeny, including major subclades. The topology of this phylogeny is based on molecular sequence data. Image by Jonathan R. Hendricks.
The following chapters of this textbook will introduce you in greater detail to each of these major clades, as well as their constituent phyla, classes, and other subgroupings. Understanding which features both unite (synapomorphies) and differentiate the phyla is useful for both understanding them biologically, as well as for recognizing their evolutionary history.
References and further reading
Aguinaldo, A.M.A., J.M. Turbeville, L.S. Linford, M.C. Rivera, J.R. Garey, R.A. Raff, and J.A. Lake. 1997. Evidence for a clade of nematodes, arthropods and other moulting animals. Nature 387: 489–493.
Field, K.G., G.J. Olsen, D.J. Lane, S.J. Giovannoni, M.T. Ghiselin, E.C. Raff, N.R. Pace, and R.A. Raff. 1988. Molecular phylogeny of the animal kingdom. Science 239: 748–753.
Goulding, M.Q. 2009. Cell lineage of the Ilyanassa embryo: evolutionary acceleration of regional differentiation during early development. PLoS ONE 4(5): e5506.
Halanych, K.M. 2004. The new view of animal phylogeny. Annual Review of Ecology, Evolution, and Systematics. 35: 229–256.
Halanych, K.M., J.D. Bacheller, A.M. Aguinaldo, S.M. Liva, D.M. Hillis, and J.A. Lake. 1995. Evidence from 18S ribosomal DNA that the lophophorates are protostome animals. Science 267: 1641–1643.
Kozloff, E.N. 1990. Invertebrates. Saunders College Publishing, Philadelphia, 866 pp.
Larsson, A. I., J. Järnegren, S.M. Strömberg, M.P. Dahl, T. Lundälv, and S. Brooke. 2014. Embryogenesis and larval biology of the cold-water coral Lophelia pertusa. PLoS ONE 9(7): e102222.
Telford, M.J., G.E. Budd, and H. Philippe. 2015. Phylogenomic insights into animal evolution. Current Biology 25, R876–R887.
Content usage
Usage of text and images created for DEAL: Text on this page was written by Jonathan R. Hendricks. Original written content created by Jonathan R. Hendricks for the Digital Encyclopedia of Ancient Life that appears on this page is licensed under a Creative Commons Attribution-NonCommercial-ShareAlike 4.0 International License. Original images created by Jonathan R. Hendricks are also licensed under Creative Commons Attribution-NonCommercial-ShareAlike 4.0 International License.
Content sourced from other websites: Attribution, source webpage, and licensing information or terms of use are indicated for images sourced from other websites in the figure caption below the relevant image. See original sources for further details. Attribution and source webpage are indicated for embedded videos. See original sources for terms of use. Reproduction of an image or video on this page does not imply endorsement by the author, creator, source website, publisher, and/or copyright holder.