Page by:
Jonathan R. Hendricks, Paleontological Research Institution, Ithaca, New York
This page was first publicly announced on May 29, 2020.
Chapter contents:
Chordata: Overview and Basal Taxa
- Jawless Vertebrates ←
- Note: Additional pages are in preparation.
Topics covered on this page: Overview; Cyclostomes; Conodonts; Ostracoderms; and Summary.
Image above: Conodont elements that have been experimentally subjected to different amounts of heat. Image is from fig. 4 in Epstein et al. (1977) (public domain).
Overview
Traditionally, the name "Agnatha" was used to characterize basal vertebrates that lack jaws (the word Agnatha is Greek for "no jaws"). The group, however, is paraphyletic because it does not include the monophyletic gnathostomes (vertebrates with jaws, ranging from sharks to humans). As such, this assemblage of animals will be referred to here simply as "jawless vertebrates." The vast majority of jawless vertebrate diversity (mostly represented by ostracoderm fishes and conodonts) is now extinct, but one clade—the eel-shaped cyclostomes (hagfish and lampreys)—survive to the present day.
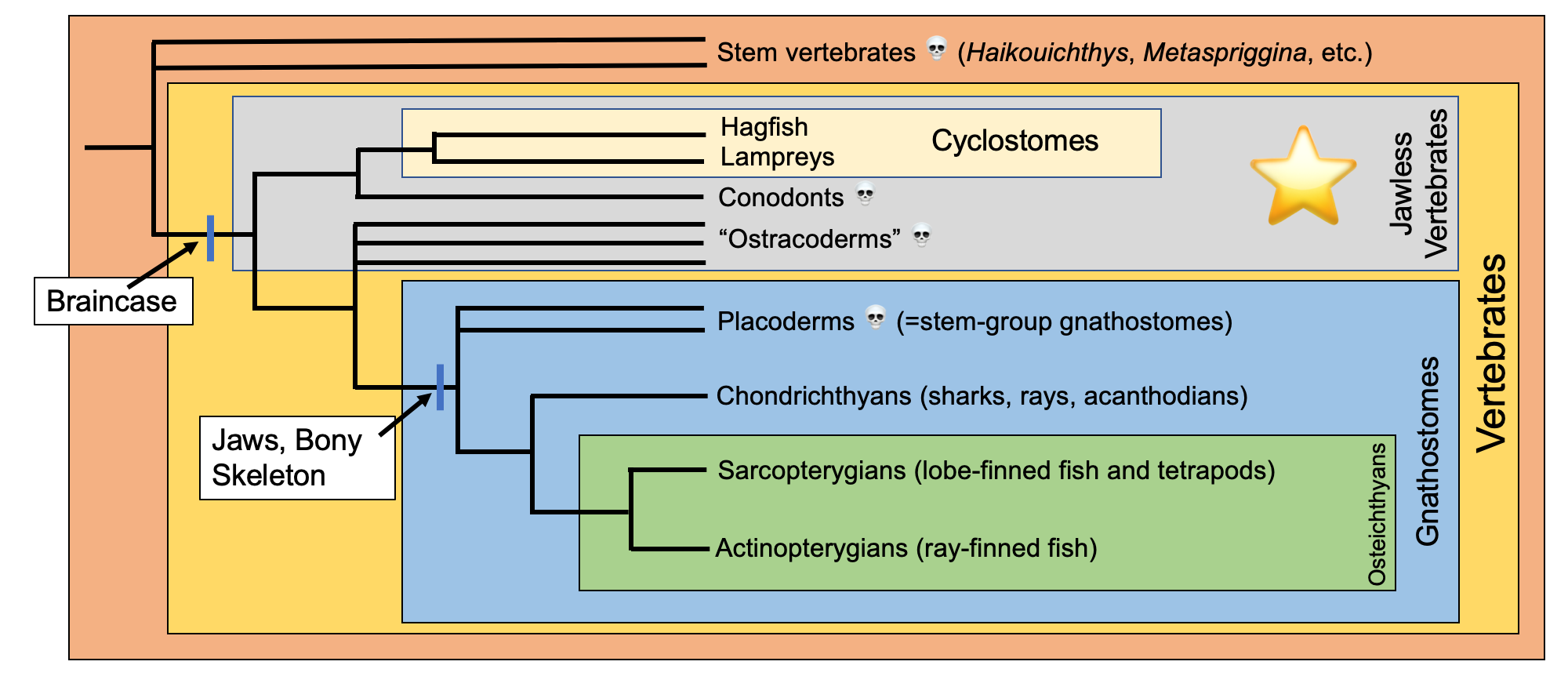
Simplified overview of vertebrate phylogeny. The jawless vertebrates (traditionally recognized as "agnathans") are identified by the gray box and star. This tree was compiled using several recent publications, including Brazeau and Friedman (2015), Brazeau and de Winter (2015), Janvier (2015), Janvier and Sansom (2016), Donoghue (2017), Miyashita et al. (2019). Image by Jonathan R. Hendricks.
Cyclostomes: Hagfish and Lampreys
Cyclostomes are a monophyletic group of eel-shaped animals that include modern hagfish and lampreys. Members of both groups have cartilaginous skulls, qualifying them as true crown-group vertebrates, but lack jaws. In fact, they are the only two groups of extant vertebrates that lack jaws.
Hagfish
In the opinion of the author, few living animals are grosser than hagfish. These marine animals are opportunistic and feed on invertebrates and the decaying bodes of much larger animals, including deceased whales. They use teeth attached to an extrudable plate to rasp and pull flesh from their food.
"The Hagfish is the Slimy Sea Creature of Your Nightmares" by Smithsonian Channel (YouTube).
To make matters even more disgusting, they are renowned for producing copious (accounts almost always use that word) amounts of slime as a deterrent against their own would-be predators.
"Eddie and the Hagfish" by Mary Lynn Price (YouTube).
Hagfish, also called "slime eels" for obvious reasons, made national news in 2017 when a truck carrying hundreds of them crashed on Highway 101 in Oregon. The results were, predictably, very slimy. (Learn more about hagfish slime in this recent article by Ed Yong.)
"Truck full of slime eels crashes on Highway 101" by KGW8 News (YouTube).
Hagfish have a very poor fossil record. A handful of taxa have been described from the Carboniferous period (e.g., see taxa described by Bardack and Richardson, 1977), but the exact relationships of these fossils to crown-group hagfish have proven somewhat controversial. Miyashita et al. (2019), however, recently published a Late Cretaceous specimen from Lebanon that they characterize as "an unequivocal fossil hagfish" (p. 2146).
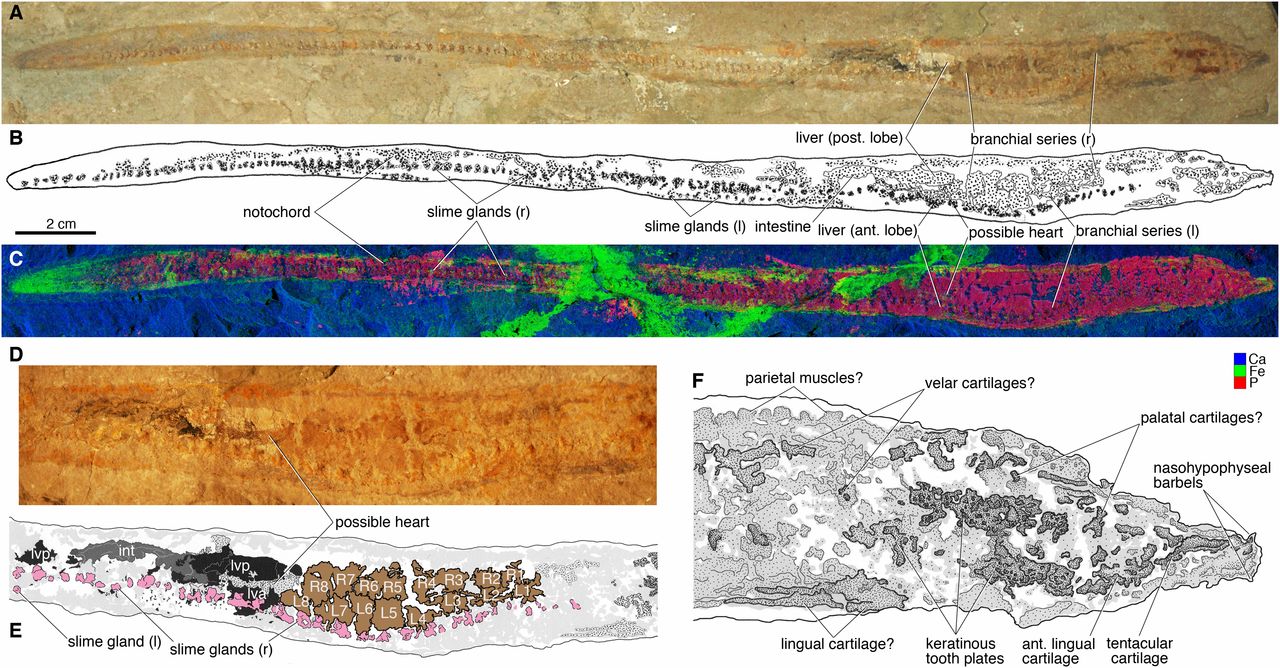
Fossil hagfish from the Cretaceous of Lebanon. Image is from fig. 2 in Miyashita et al. (2019) in PNAS (Open Access; Creative Commons Attribution License 4.0). Original caption: "Tethymyxine tapirostrum gen. et sp. nov, a fossil hagfish from the Cenomanian of Lebanon. Holotype (BHI 6445) in right lateral view: (A) photograph; (B) interpretive drawing; (C) false-color composite of distributions of three selected chemical elements (blue = Ca; green = Fe; red = P; Ca and Fe in HZ setup and P in LZ setup, 99.9% threshold) from SRS-XRF. The visceral anatomy of BHI 6445 in composite photograph (D) and interpretive drawing (E) in the following color codes: black = liver lobes; brown = branchial pouches; dark gray = intestine; light gray = preserved amorphous tissues; pink = slime glands; stippled gray = other soft tissues that are preserved with distinct outlines. The cranial anatomy of BHI 6445 in interpretive drawing (F) in which preserved structures are indicated in gray shades and stipples. Abbreviations: ant, anterior; int, intestine; L, left branchial pouch; l, left side; lva, liver, anterior lobe; lvp, liver, posterior lobe; post, posterior; R, right branchial pouch; r, right side."
As part of their study of this new fossil, Miyashita et al. (2019) conducted a detailed phylogenetic analysis that included modern hagfish, lamprey, and other fish, as well as a diversity of extinct taxa, including some of the stem vertebrates mentioned on the previous page (e.g., Haikouichthys and Metaspriggina), conodonts (see below), and a variety of jawless fishes. Reinforcing an earlier finding by Heimberg et al. (2010), they found that hagfish and lampreys form a monophyletic group (Cyclostomi) (overturning an earlier idea that lampreys are more closely related to jawed vertebrates than they are to hagfish) and also showed that conodonts are the sister taxon of Cyclostomi.
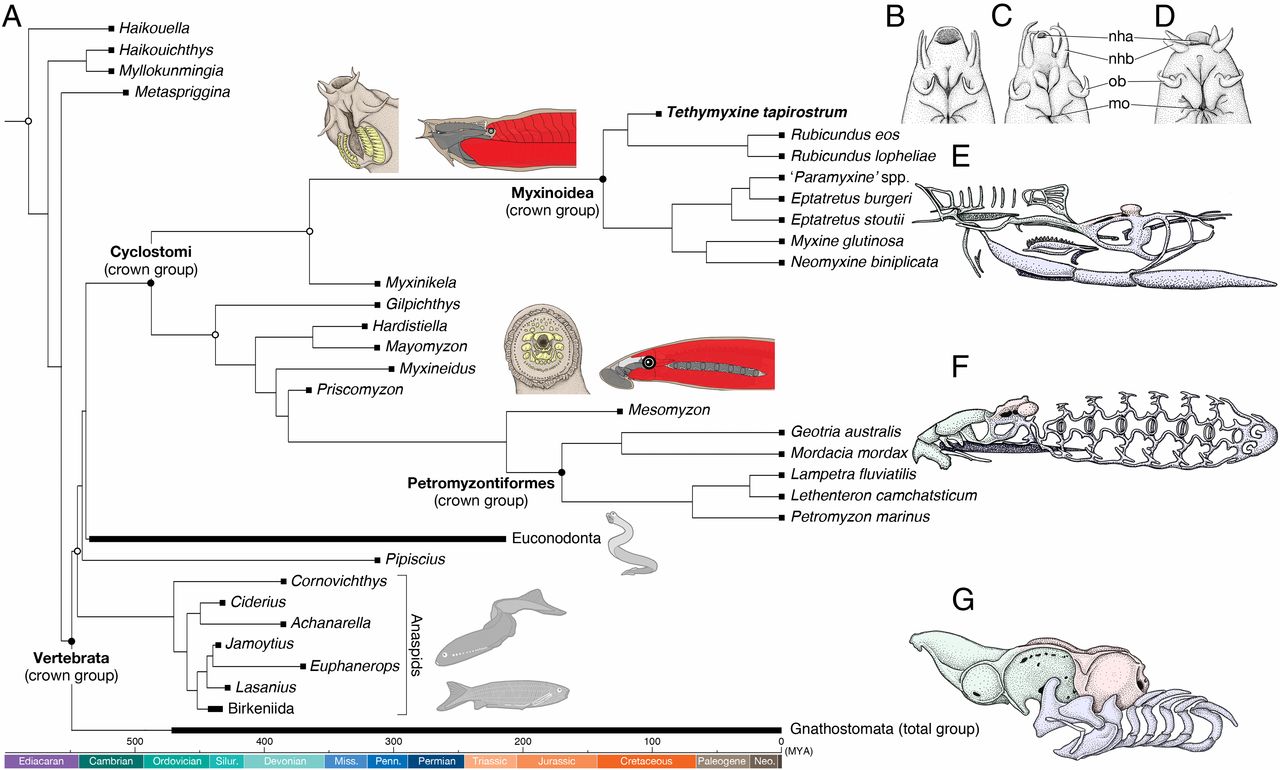
Phylogenetic relationship of cyclostomes to other vertebrates, including details of the cartilaginous skulls of hagfish and lampreys. Note also the basal positions of the stem vertebrates mentioned above. Image is from fig. 3 in Miyashita et al. (2019) in PNAS (Open Access; Creative Commons Attribution License 4.0). Original caption: "A time-scaled phylogenetic tree of cyclostomes. (A) Summary tree showing cyclostome relationships. Maximum parsimony and Bayesian inferences converged onto each other in placing Tethymyxine within the hagfish crown group and supporting cyclostome monophyly. The precise topology is from the maximum parsimony analysis (SI Appendix, Fig. S6). Node ages represent median of 95% HPD distribution in a Bayesian molecular clock analysis of mitogenomic sequences (16S and COI) under fossilized birth–death model (see SI Appendix, Fig. S9 for a fully calibrated maximum clade credibility tree with node intervals). The crown group of cyclostomes is united by at least two morphological characters (shown on each stem): keratinous tooth plates (yellow) and periocular position of trunk muscles (red). At Top Right, the nasohypophyseal profiles are compared in ventral view among three selected crown-group hagfishes (B: Tethymyxine tapirostrum; C, Rubicundus eos; D, Eptatretus stoutii; the latter two based on ref. 29). To show morphological divergence among the three major crown groups of living vertebrates (E, myxinoids; F, petromyzontiforms; G, gnathostomes), each is accompanied by a chondrocranium in left lateral view (green: neural crest-derived nasohypophyseal skeleton; red: mesodermally derived neurocranium; blue: neural crest-derived pharyngeal skeleton). Filled squares represent occurrences of the terminal taxa. Crown nodes are each indicated by a filled circle, and total nodes by an empty circle. Abbreviations: nha, nasohypophyseal aperture; nhb, nasohypophyseal barbels; mo, mouth; ob, oral barbels."
Lampreys
Like hagfish, lampreys have an eel-like shape, are similarly jawless, and also have a cartilaginous skull. Lamprey have a sucker-like mouth with many sharp teeth. Some species are ectoparasites of fish and use their mouth to latch onto their prey. The sharp tongue of the lamprey is then used to burrow through the flesh of the prey, opening a channel that the lamprey can use to feed on its blood. Lampreys are invasive species in the Great Lakes, where they have no predators, and have severely impacted some fisheries.
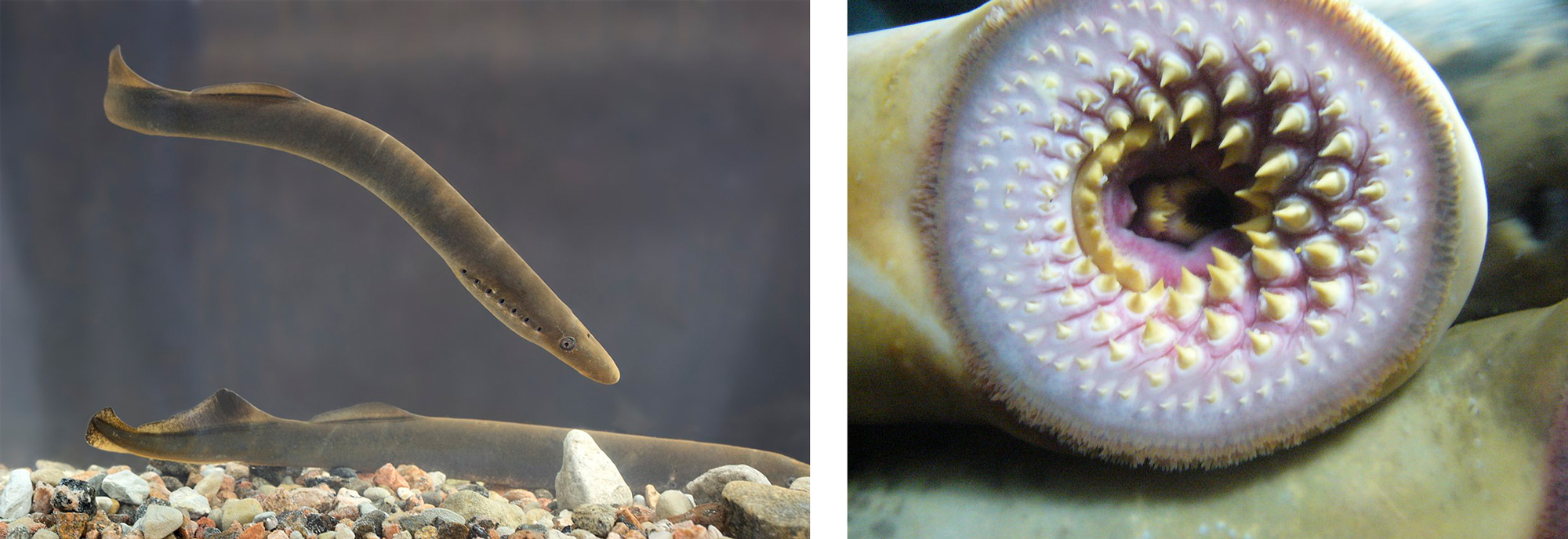
Left: Two individuals of the lamprey Lampetra fluviatilis; image by Tiit Hunt (Wikimedia Commons; Creative Commons Attribution-Share Alike 3.0 Unported license). Right: Mouth of the sea lamprey Petromyzon marinus; image by "Drow_male" (Wikimedia Commons; Creative Commons Attribution-Share Alike 3.0 Unported license).
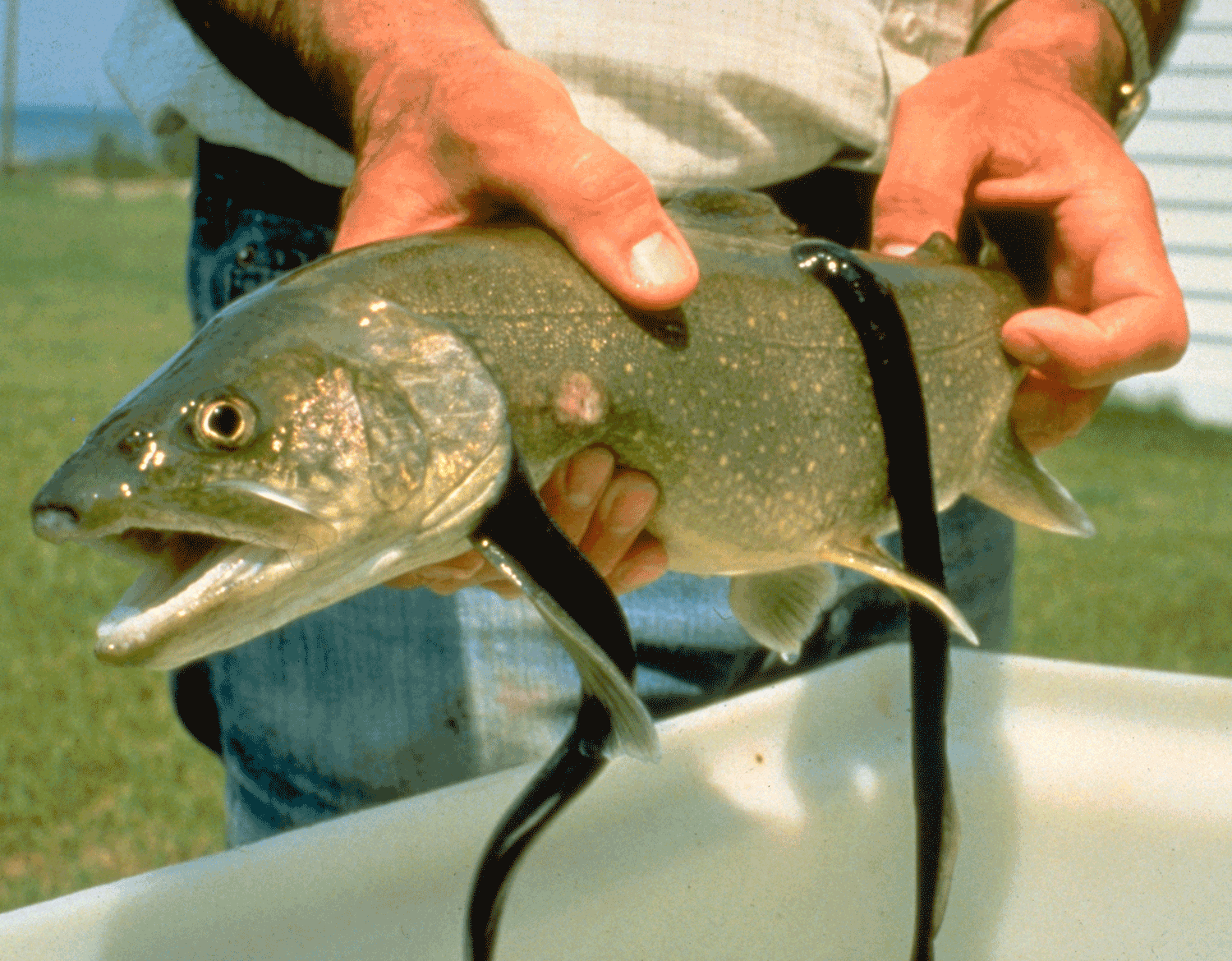
Two lampreys attached to a lake trout; image by the Great Lakes Fishery Commission (Wikimedia Commons; public domain).
"Best of Lampreys - River Monsters" by River Monsters (YouTube).
A small handful of lamprey specimens are known from the fossil record. The oldest is from the Devonian of South Africa (Gess et al., 2006) and is very similar in appearance to modern species, suggesting that lampreys are good examples of living fossils that have not changed much over hundreds of millions of years. More recently, multiple lifecycle stages (including larval forms) have been documented from the Cretaceous of Mongolia (Chang et al., 2014).
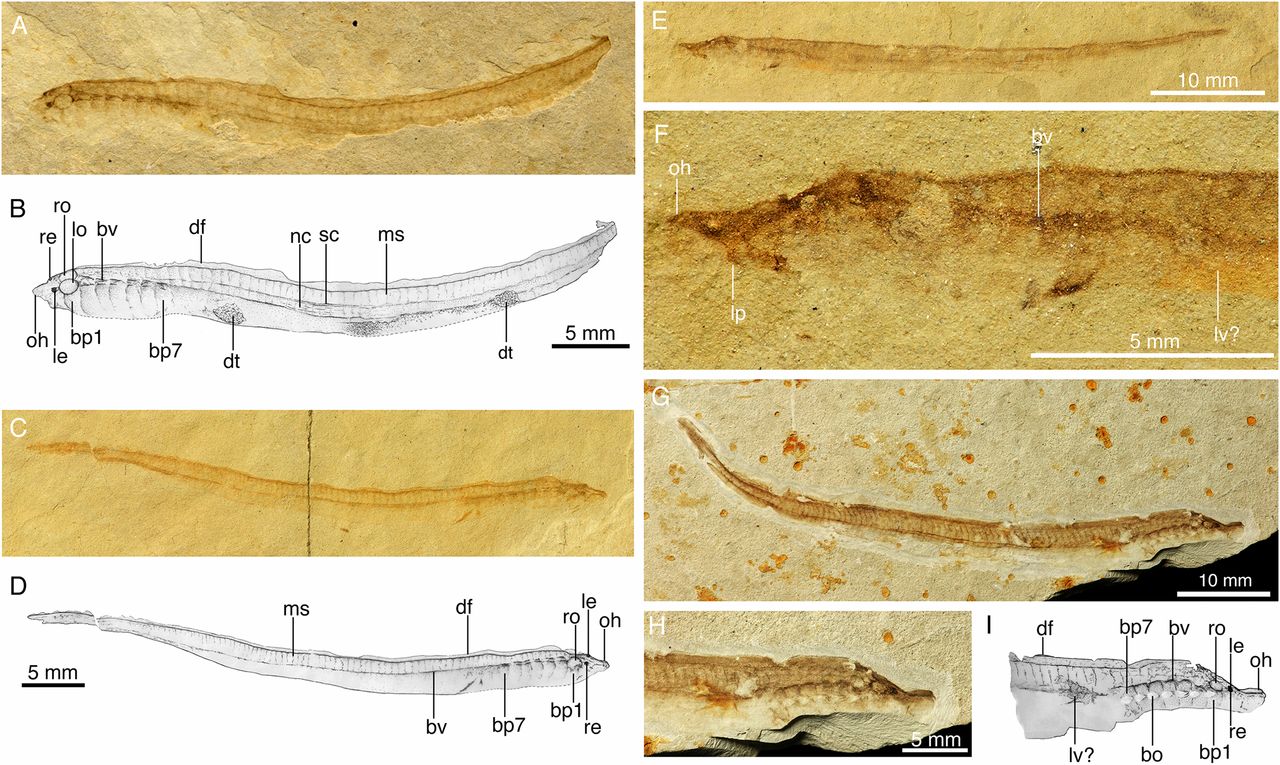
Fossils of lamprey larvae from the Cretaceous of Mongolia. Image is fig. 1 from Chang et al. (2014) in PNAS, which allows for re-use of images for educational purposes (see here). Original caption: "Larvae of M. mengae from the Lower Cretaceous of China. These 125-My-old ammocoetes are the earliest known examples of lamprey larvae in the fossil records, showing almost identical anatomy and life cycle with their living counterparts. (A) Photograph and (B) drawing of IVPP V15114.6A in left view. (C) Photograph and (D) drawing of IVPP V15165.2A in right view. (E) Photograph of in IVPP V15114.5B in left view and (F) that of the head and anterior part of the body of E. (G) Photograph of IVPP V15681A in right view. (H) Photograph and (I) drawing of head and anterior part of body of G. (bv, blood vessels; cf, caudal fin; df, dorsal fin; dt, detrital remains in digestive tract; bo, external branchial opening; bp1, bp7, first and seventh branchial pouch; le, left eye; lo, left otic capsule; lp, lower lip; lv?, possible remains of liver; ms, myoseptum; nc, notochord; oh, oral hood; re, right eye; ro, right otic capsule; sc, spinal cord)."
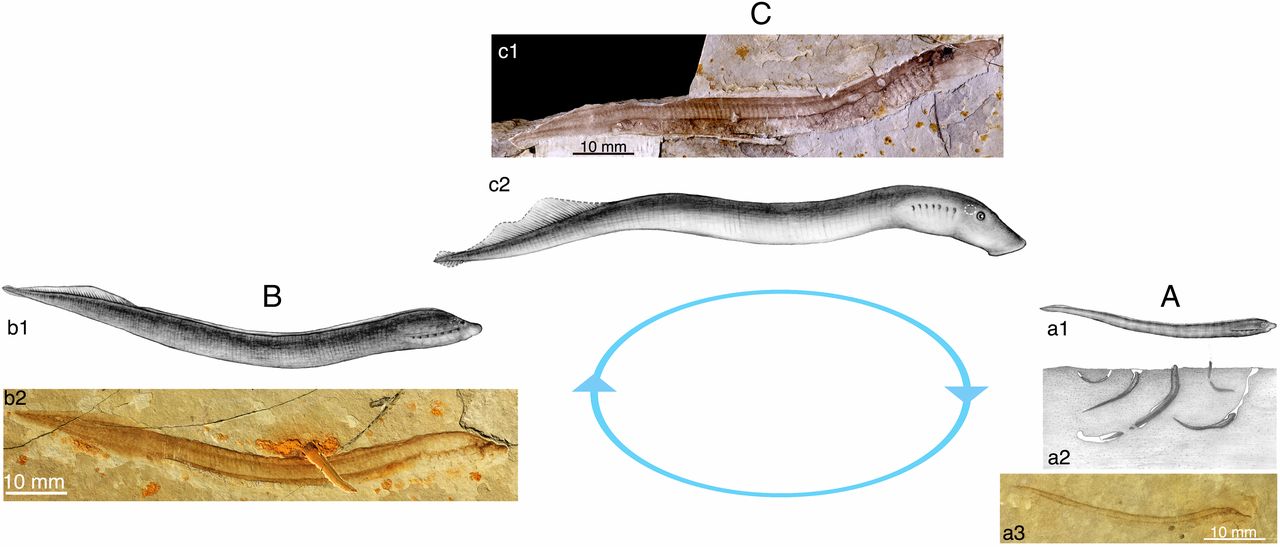
Larval and adult fossil lamprey from the Cretaceous of Mongolia. Image is fig. 3 from Chang et al. (2014) in PNAS, which allows for re-use of images for educational purposes (see here). Original caption: "Specimens and restorations of larva, early transformer, and adult of M. mengae, showing its three-phased life cycle, which emerged at least 125 Ma. (A) Larva (ammocoete) of M. mengae. a1, restoration of larval M. mengae; a2, restoration of burrowing larvae of M. mengae (cf. fig. 4.1 in ref. 7, not to scale); a3, photograph of IVPP V15034B in right view. (B) Early transformer of M. mengae. b1, restoration; b2, photograph of IVPP V15506B in right view (original photo horizontally flipped). (C) Adult or late transformer of M. mengae. c1, photograph of holotype (IVPP V14719) of M. mengae in right view; and c2, its restoration. (the circle in white dotted line in a1, b1, and c1 representing the position of otic capsule)."
Conodonts
"With just a bit of preparation, almost any marine rock of Paleozoic or Triassic age, from almost anywhere on earth, will yield to the patient investigator an assortment of phosphatic microfossils termed conodonts." - Walter Sweet (1988)
Conodonts are small, tooth-shaped fossils found in Upper Cambrian to Triassic-aged marine rocks. Such conodont elements have a great diversity of shapes and have been proven to be amongst the most important fossils for Paleozoic and Triassic biostratigraphy. This is because they check many of the boxes for being ideal index fossils: they have short durations, they are widespread, and many are morphologically complex.
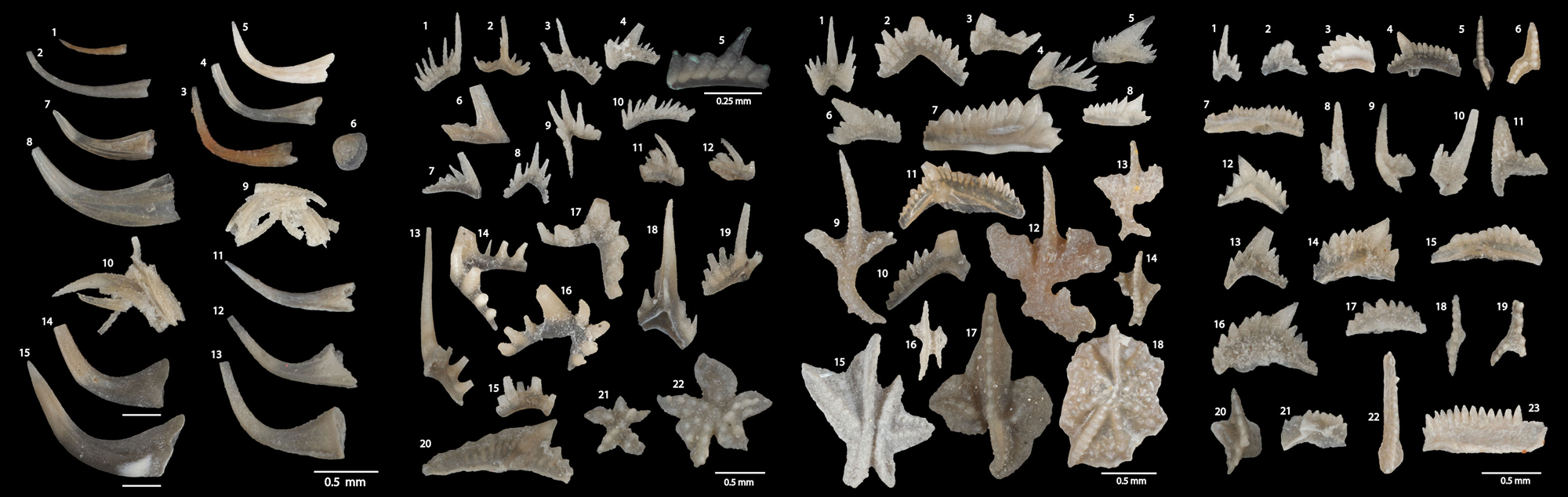
Examples of conodont elements from the Silurian of eastern Iowa. Images are from figs. 7-10 (combined here) in Waid and Cramer (2017; Palaeontologia Electronica); Creative Commons Attribution-NonCommercial-ShareAlike 4.0 International license.
Conodont elements vary significantly in their complexity. Coniform elements are cone shaped and consist of a base and single cusp. Ramiform elements are blade-shaped and have an elongated cusp that is surrounded by or adjacent to shorter denticles. Pectiniform elements have lateral extensions (which may be broad) called platforms; multiple ridges are sometimes present that are covered with denticles.
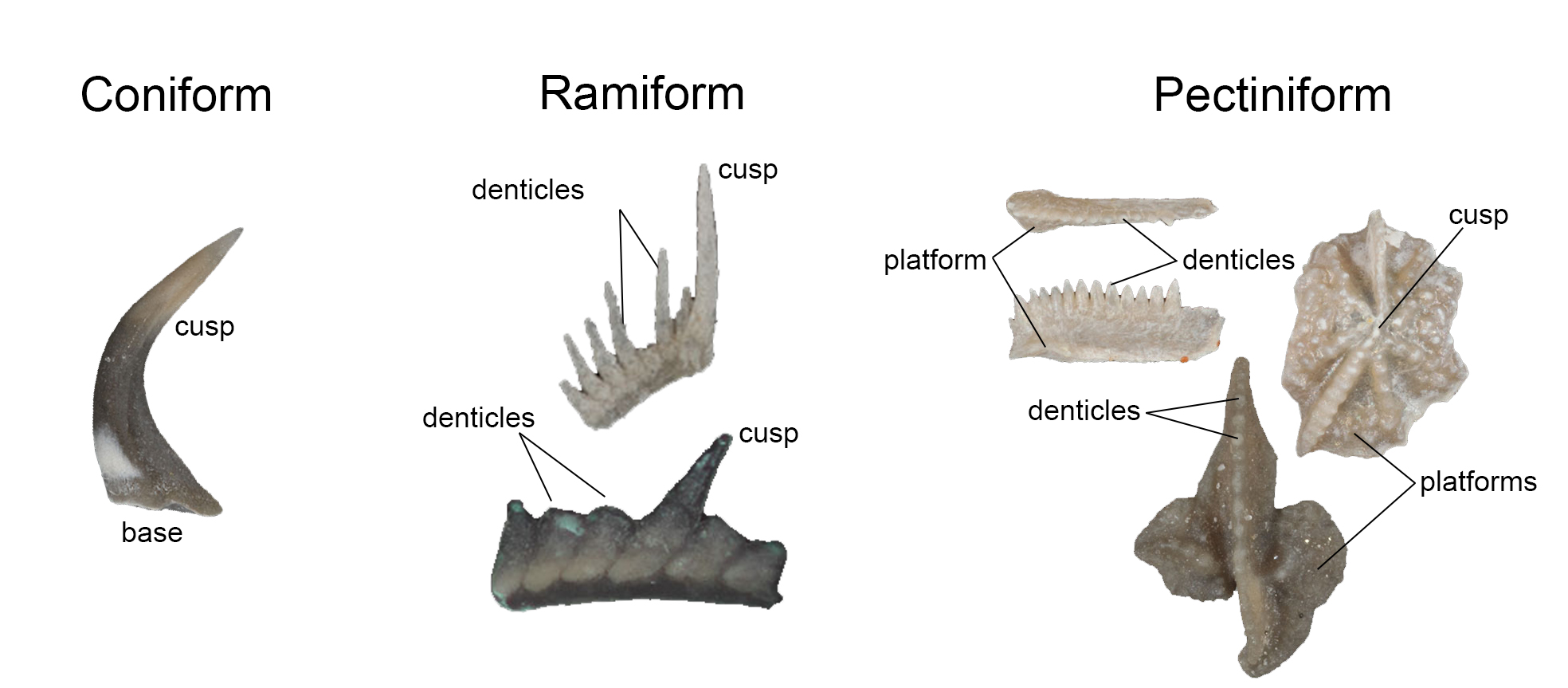
The three most basic forms of conodont elements: coniform, ramiform, and pectiniform. Images of individual conodont elements are from figures published in Waid and Cramer (2017; Palaeontologia Electronica); Creative Commons Attribution-NonCommercial-ShareAlike 4.0 International license. Image constructed by Jonathan R. Hendricks.
Besides being very important for correlating Paleozoic rocks and determining their ages, conodonts are also very useful for determining the extent to which rocks have been heated within the Earth. Significantly, conodont elements, which are composed of the phosphatic mineral hydroxyapatite, change color as they are heated. By analyzing their color, researchers are able to determine the extent to which surrounding rocks have been "cooked" within Earth's crust. This in turn is useful for determining whether hydrocarbons have been "cooked" long enough to be worth extracting for energy. The discovery that conodont elements change color when heated was made and pioneered by Dr. Anita [Epstein] Harris (1937-2014), who worked for the United States Geological Survey. Through her research, Harris developed the conodont color alteration index (CAI) (Epstein et al., 1977), which proved to be very useful for both understanding the regional thermal histories of rocks and also energy exploration.
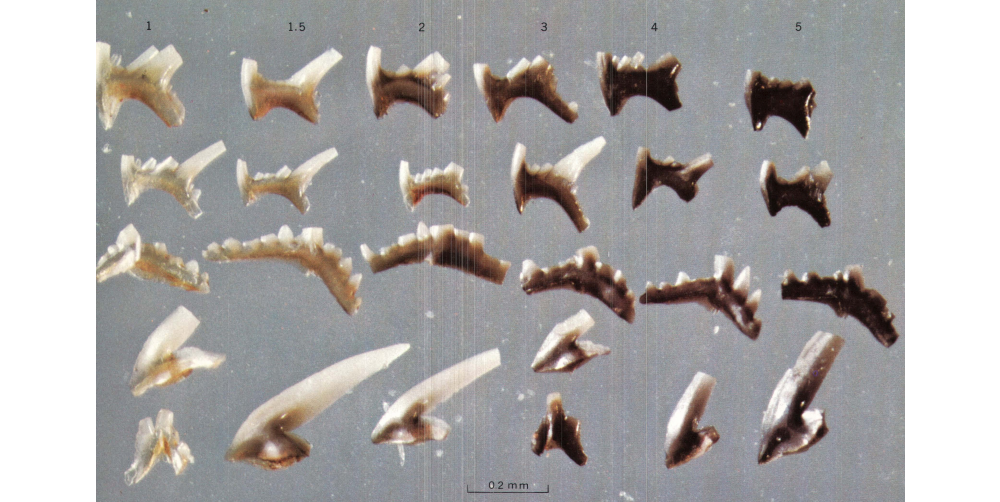
Conodont elements that have been experimentally subjected to different amounts of heat. Image is from fig. 4 in Epstein et al. (1977) (public domain). Original caption: "Late Ordovician conodont elements showing experimentally induced color alteration. CAI given at top of each column. Most of the conodonts shown are ... elements of Phragmodus undatus" (p. 5).
You now know what conodont elements look like and why they are useful for addressing geological problems, but what kind of animal did they belong to? Given their position in this chapter, you have probably already suspected (correctly!) that they are some kind of jawless vertebrate, but recognition of this is a relatively recent development. For many years, paleontologists had no idea what the conodont animal looked like, much less its phylogenetic affinities. All they had were scattered bits and pieces of all different shapes.
The first assemblages of multiple conodont elements, preserved in small clumps on bedding planes, were discovered in the 1930s. These assemblages showed bilaterally symmetrical pairs of different types of conodont elements. This demonstrated that not only was the conodont animal likely bilaterally symmetric, but also that a single animal possessed multiple types of conodont elements (i.e., coniform, ramiform, and pectiniform elements; see image above). Discovery of these natural assemblages led paleontologists in the 1950s and 1960s to begin making better sense of the isolated conodont elements that they had been studying. In particular, they began to recognize that certain types of elements were consistently found in the same samples because they came from individual species. Shortly thereafter, conodont students began characterizing conodont species based on multiple elements, even if natural assemblages of elements had not been found (this is called multielement taxonomy).
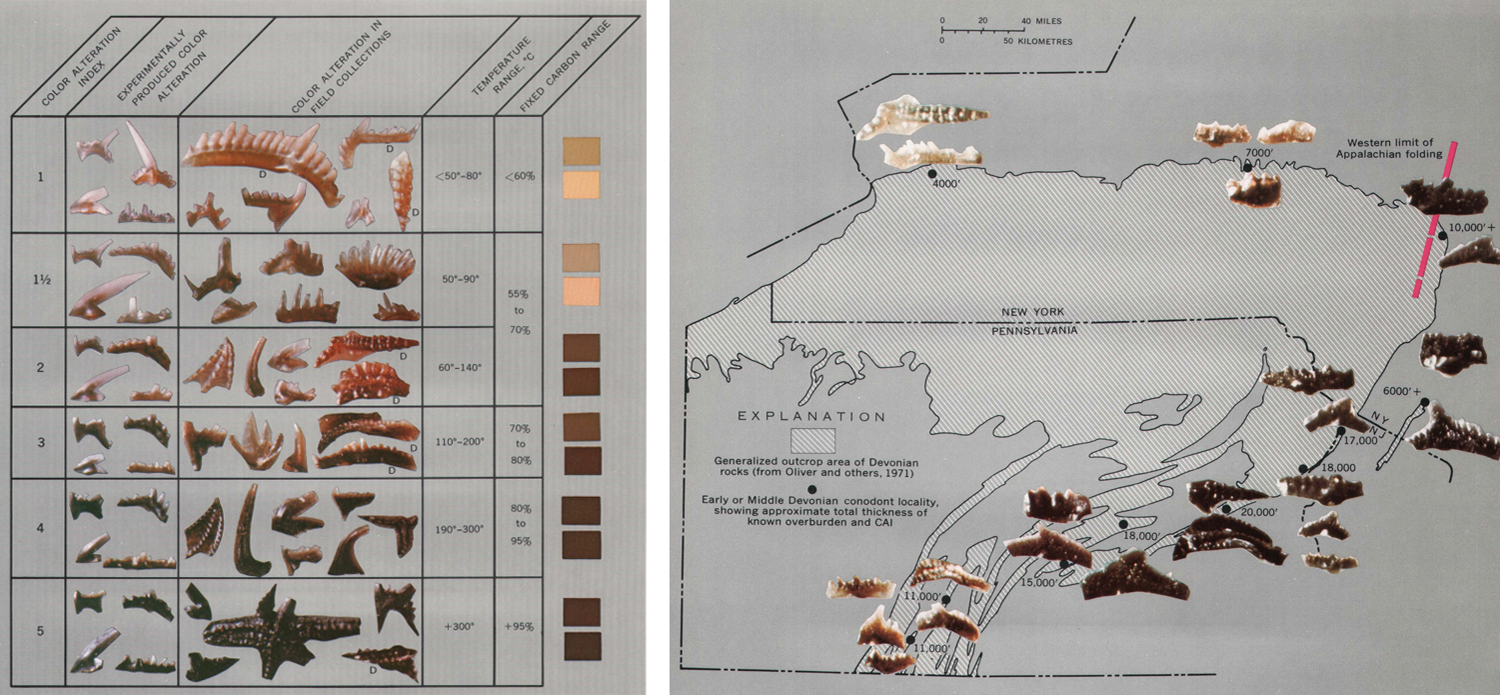
Left: The conodont color alteration index (CAI) developed by Epstein et al. (1977). Image from fig. 5 in Epstein et al. (1977) (public domain). Right: Example of how the natural color of conodont elements corresponds with their depth of burial, and therefore degree of heating. Image from fig. 10 in Epstein et al. (1977) (public domain). Original caption: "Map showing selected localities for Early and early Middle Devonian conodonts in the northeastern part of the Appalachian basin. Conodonts, CAI, and known overburden are shown for each locality. Note the correlation of conodont color (CAI) with thickness of overburden regardless of tectonic setting (compare south-central Pennsylvania with central New York). The thicker the overburden, the darker the conodont!" (p. 12).
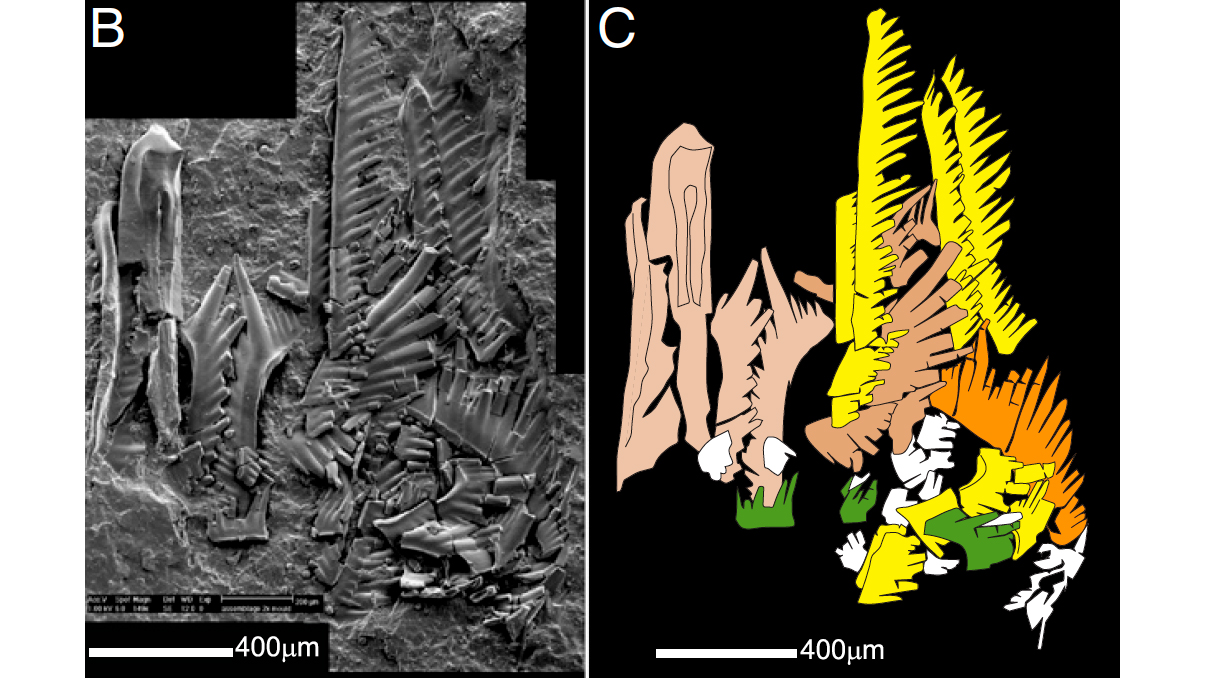
Left: scanning electronic microscope image of a natural assemblage of conodont elements belonging to the Triassic genus Neogondolella; right: interpretation of the elements. Images from fig. 2 in Goudemand et al. (2011) in PNAS, which allows for re-use of images for educational purposes (see here). Original caption: "(B) SEM composite microphotograph and (C) interpretation of a natural assemblage of Neogondolella found by Rieber (also ref. 16) from the Middle Triassic at Monte San Giorgio, Switzerland. Beige: P elements; orange: S0; brown: S1 and S2; yellow: S3 and S4; green: M elements" (p. 8721).
Modern condont workers now characterize individual condont elements based on where they are positioned in the conodont element "apparatus," which was first determined based on the discovery of natural assemblages. The bilaterally-symmetrical apparatus includes 15-19 individual elements that are divided among three distinct structural regions called S, M, and P (Sweet and Donoghue, 2001). Sweet and Donoghue (2001) characterized these S-, M-, and P-region elements as follows:
- S-region elements: "one element is itself bilaterally symmetrical and unpaired; the remainder are fragile, paired, profusely denticulated structures, elongate antero-posteriorly" (p. 1179). These elements are ramiform in shape.
- M-region elements: "a pair of pick-shaped elements, commonly with one or two long denticulated processes, is situated outboard of the S group" (p. 1179).
- P-region elements: "were commonly the stoutest, were approximate mirror images of one another, had their long axes oriented dorso-ventrally and their denticulated surfaces opposed" (p. 1179). These elements are pectiniform in shape.
A 3D model showing a reconstruction of a conodont element apparatus (with S-, M-, and R-region elements identified) is shown below.
3D model of the conodont element apparatus of Kladognathus sp. S-, M-, and P-elements are identified with notations. Model by "micropaleontology" (Sketchfab).
Some conodont species had apparatuses that were apparently composed only of simple, coniform elements. Sweet and Donoghue (2001) noted that we know very little about natural assemblages associated with such species and that it is not possible to fit their coniform elements into the S M P scheme described above, much less make definitive statements about their homology with S M P elements.
The "eureka moment" for understanding the phylogenetic affinities of conodonts came in the early 1980s when a remarkable soft-bodied fossil from the Carboniferous of Scotland was discoverd that showed an assemblage of conodonts elements positioned in the head region of a small, big-eyed, eel-like animal. Importantly, the specimen also showed V-shaped muscles called myomeres that are present in fish, as well as evidence of a notochord. These features have convinced most paleontologists that condonts were vertebrates, as had long been suspected based on the phosphatic hydroxylapatite mineral composition of the elements themselves: this is the same mineral used by vertebrates to build bone. The conodont animal had finally been discovered and was published by Briggs et al. in 1983. Following this discovery, a small handful of additional soft-bodied specimens of condont have been discovered.
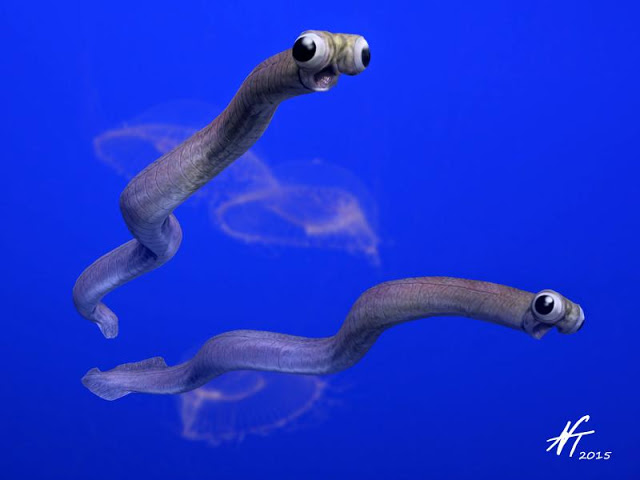
Reconstruction of the Mississippian conodont Clydagnathus cavusformis by Nobu Tamura (Creative Commons Attribution-Share Alike 4.0 International license).
Establishing that conodont elements were associated with the head of the animal allowed paleontologists to begin to try to understand how they functioned, whether for grasping prey or some other purpose, and research in this area continues. The video below shows a reconstruction of how conodont elements may have moved relative to each other in the mouth of the Triassic species Novispathodus (see image above of the natural assemblage of conodont elements from which this model was developed).
"Feeding Mechanism of Conodonts" by UniversitaetZuerich (Youtube) based on work published by Goudemand et al. (2011 in PNAS).
The exact relationship of conodonts to living vertebrates remains contentious, owing in part to the very few number of body fossils discovered that provide information about their soft-body anatomy. Some researchers have found them to be the sister taxon of cyclostomes (which include hagfish and lampreys; see phylogeny at the top of this page), while others have found them to be stem-group gnathostomes (though they lacked jaws). Regardless, they remain a very important group of fossils for addressing geological problems, from determining the ages of rocks (biostratigraphy) to exploring for oil and gas. The video below summarizes some of the material reviewed above and also shows some examples of major boundaries in the geological time scale that are based on conodont biostratigraphy (e.g., the Devonian-Carboniferous boundary).
"The Most Useful Fossils in the World" by PBS Eons (YouTube).
"Ostracoderms"
Ostracoderms are an extinct, paraphyletic grouping of jawless fishes that were characterized by a bizarre diversity of bony head shields. They were the most important vertebrates for nearly 100 million years prior to the origin of their descendants, the jawed gnathostomes. As noted by Donoghue and Keating (2014), "ostracoderm lineages are related by degree to the living jawed vertebrates ... betrayed by the hierarchically nested suites of characters that they possess" and "effectively [record] the piecemeal assembly of the body plan shared by all crown gnathostomes" (p. 885).
The most important innovation of this group of vertebrates is the the mineralized skeleton itself. It might surprise you that we have two skeletons: an endoskleteton (most of the bones inside of our body that we typically think about) and an exoskeleton, which includes our teeth and some of the bones in our skulls. The exoskeleton, also called the dermal skeleton, of ostracoderms was much more extensive than it is in our bodies, or the bodies of most other extant vertebrates for that matter. The dermal skeleton, as its name implies, is derived from the skin (when you have problems with your skin, you go to see a dermatologist). In ostracoderms, the dermal skeleton formed not only the teeth, but also a diversity of bony scales, plates, and the fin rays still present in extant fish. Mineralized elements of the dermal skeleton consist of enamel, dentine, and bone. Where have you heard of enamel and dentine before? Probably from an advertisement for toothpaste or at your dentist's office.
There were five major groups of jawless ostracoderms, each of which is briefly introduced below.
Pteraspidomorphs
Based on the fossil record, Pteraspidomorphs originated during the Early Ordovician and were the first vertebrates to exhibit a dermal skeleton that functioned much like body armor. Their headshields were trailed by an elongate body and tail that were covered in bony scales. The headshields themselves consisted of a dorsal (top) and ventral plate (bottom).
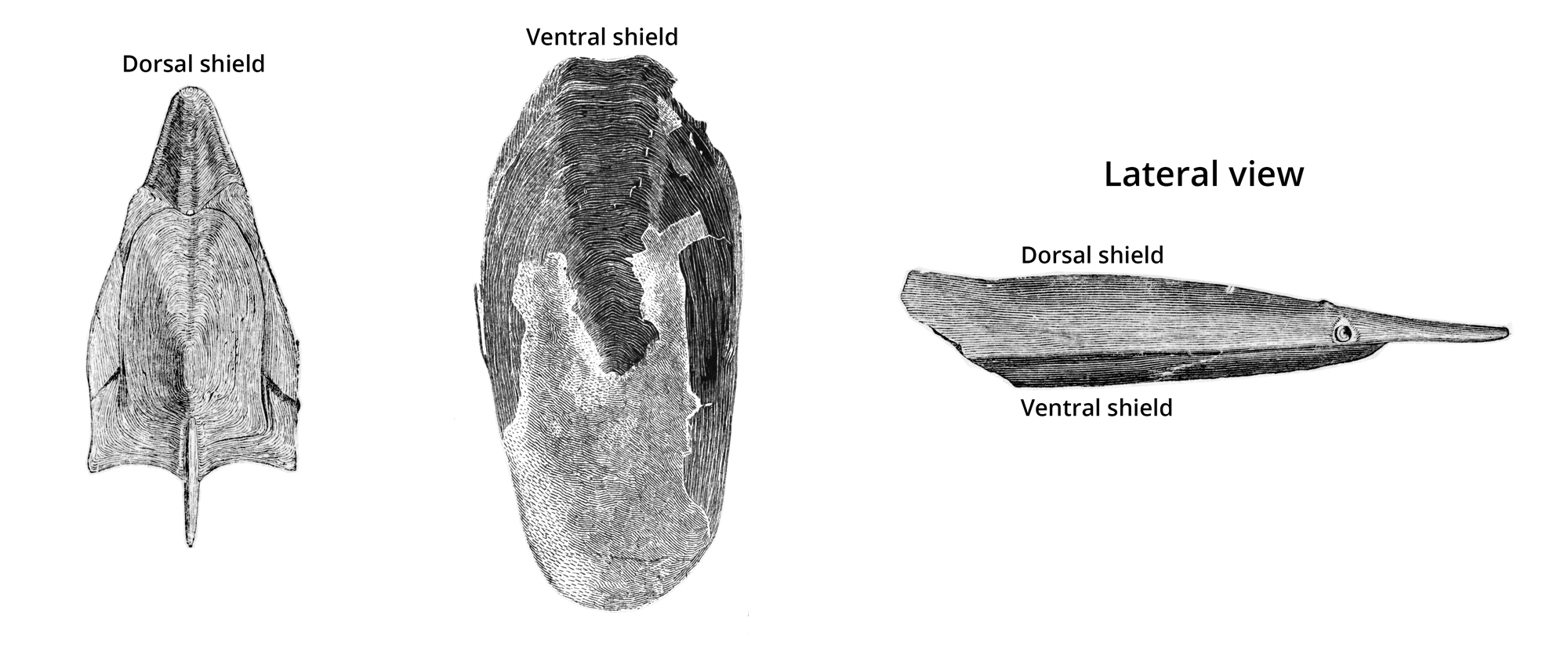
Dorsal and ventral headshields of Pteraspis; image modified from fig. 101 in Zittel (1902) (public domain).
In drastic contrast to modern fish, they lacked paired fins and had an endoskeleton that was composed of cartilage, rather than bone. In general appearance, many looked something like frog tadpoles. Three of the most significant groups of pteraspidomorphs are Arandaspida, Astraspida, and Heterostraci.
Arandaspida
Arandaspids lived during the Ordovician period. Detailed information about the group can be found on the Tree of Life website.
Arandaspis
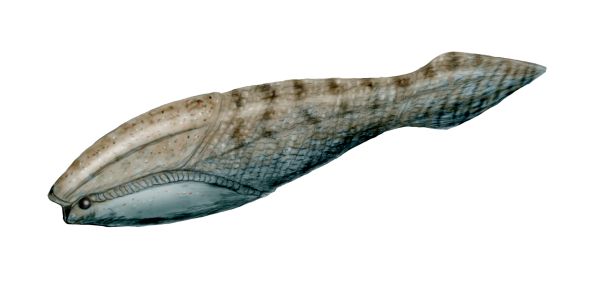
Reconstruction of the Ordovician pteraspidomorph (Arandaspida) Arandaspis prionotolepis by Nobu Tamura (Creative Commons BY-NC-ND 3.0 license).
Sacabambaspis
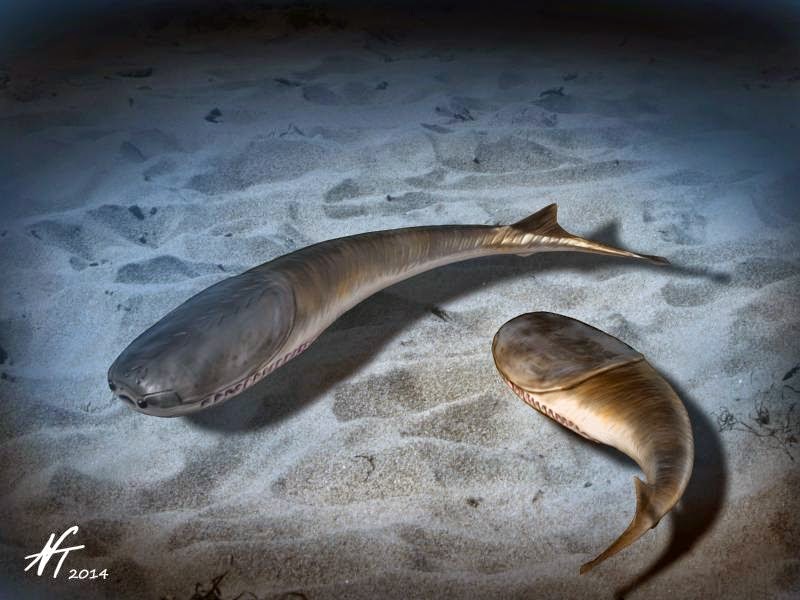
Reconstruction of the Ordovician pteraspidomorph (Arandaspida) Sacabambaspis janvieri by Nobu Tamura (Creative Commons Attribution-ShareAlike 4.0 International license).
Astraspida
Astraspids lived during the Ordovician period and are represented by only two species in the genus Astraspis. Detailed information about the group is available on the Tree of Life website.
Astraspis
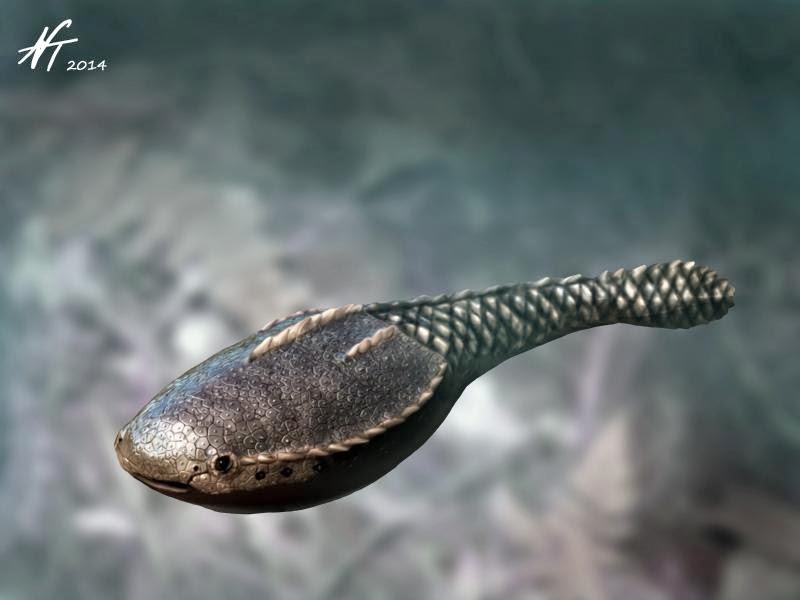
Reconstruction of the Late Ordovician pteraspidimorph (Astraspida) Astraspis desiderata by Nobu Tamura (Creative Commons Attribution-ShareAlike 4.0 International license).
Heterostraci
The largest group of pteraspidomorphs was the Heterostraci clade, which included over 300 species that lived during the Silurian and Devonian periods. They had a diversity of forms, but all exhibit one hole on either side of their head shields that likely served a resperatory function. These fish are thought to have occupied a diversity of habitats; some had flattened shapes and likely spent most of their time on the seafloor, while others have streamlined forms that suggest they were active swimmers. Detailed information about the clade can be found on the Tree of Life website.
Athenaegis
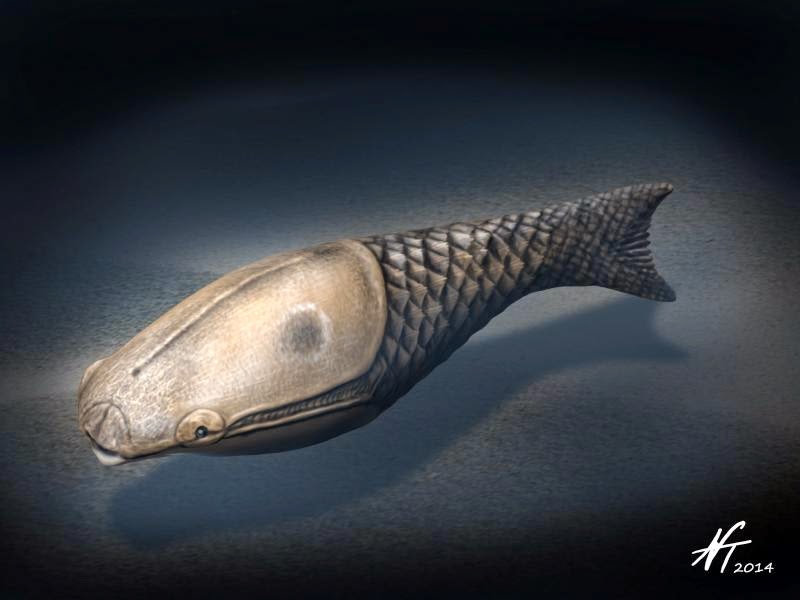
Reconstruction of the Early Silurian heterostracan fish Athenaegis chattertoni by Nobu Tamura (Creative Commons Attribution-ShareAlike 4.0 International license).
Anglaspis
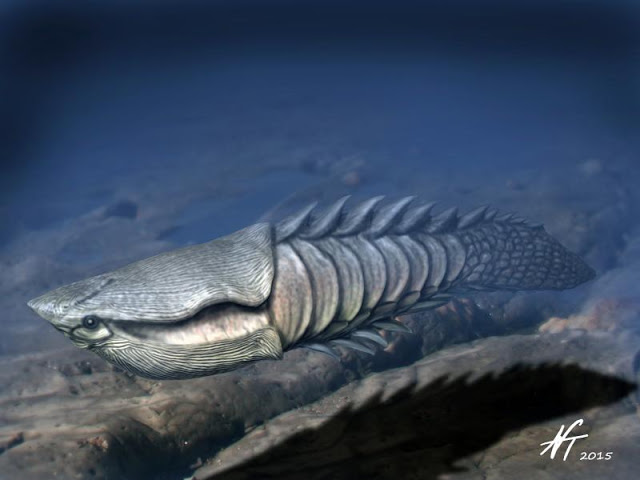
Reconstruction of the Early Devonian heterostracan Anglaspis heintzi by Nobu Tamura (Creative Commons Attribution-ShareAlike 4.0 International license).
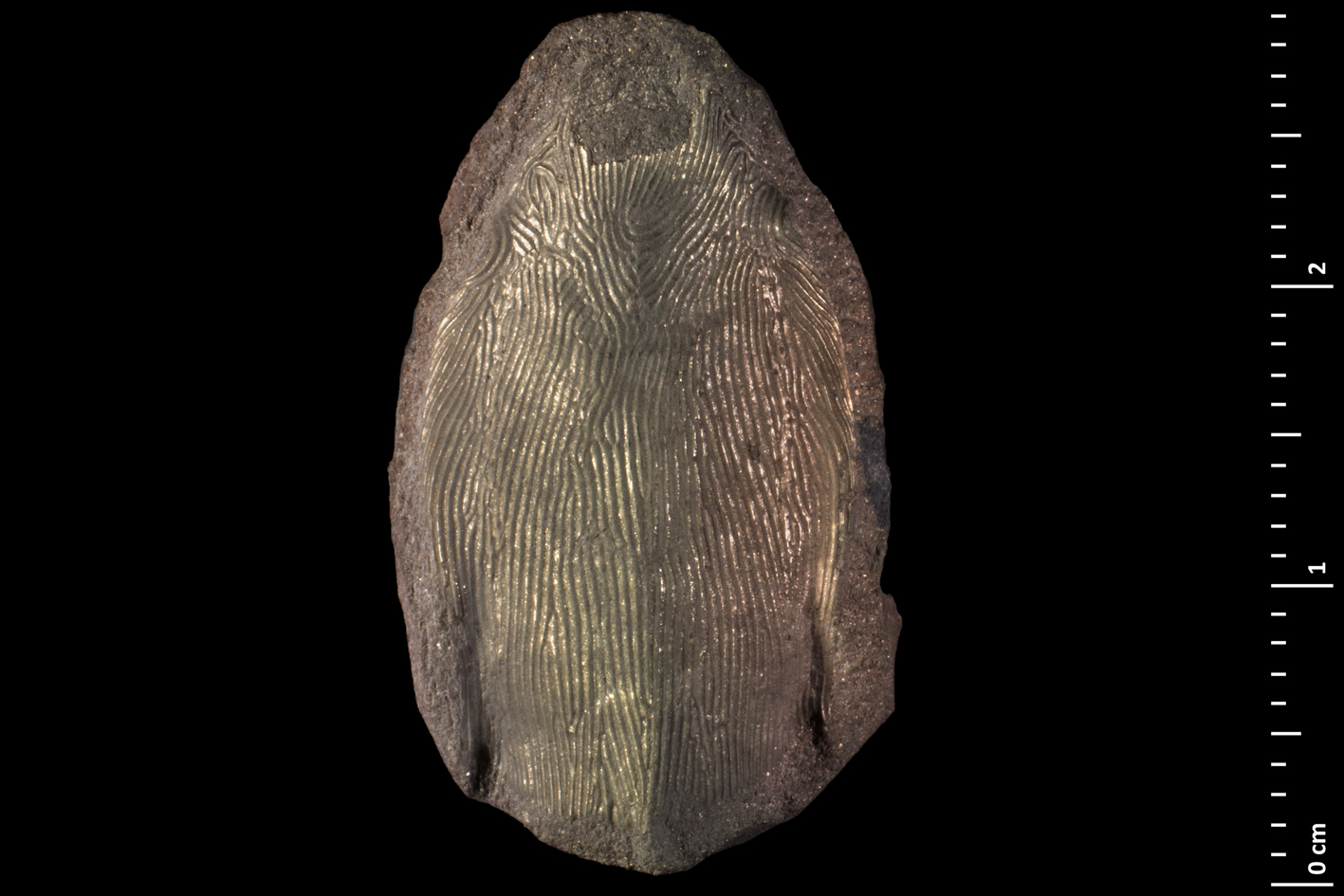
Headshield of Anglaspis heintzi from the collections of the Museum National d'Histoire Naturelle, Paris (MNHN SVD694).
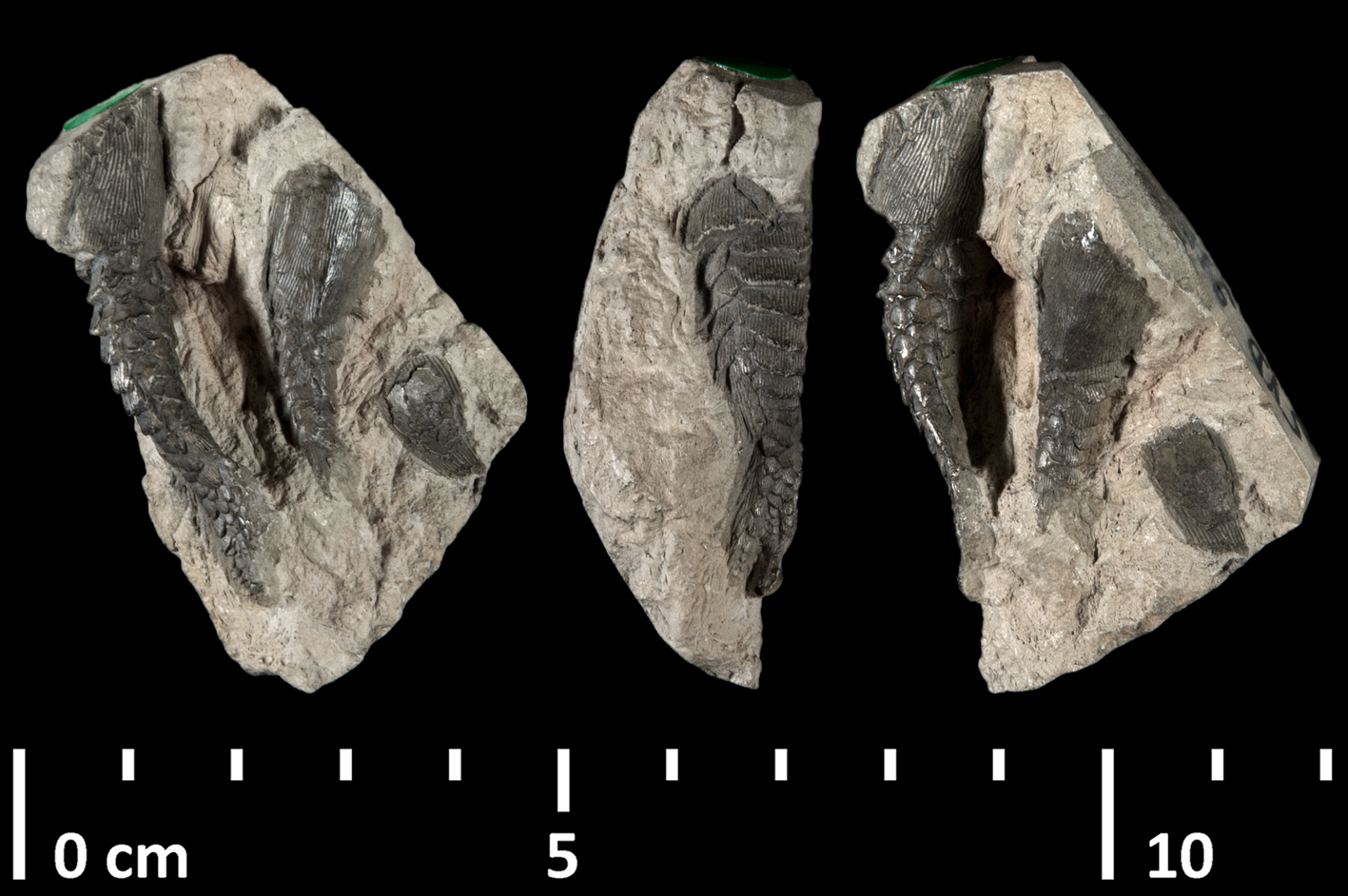
Specimens of Anglaspis heintzi from the collections of the Museum National d'Histoire Naturelle, Paris (MNHN SVD911).
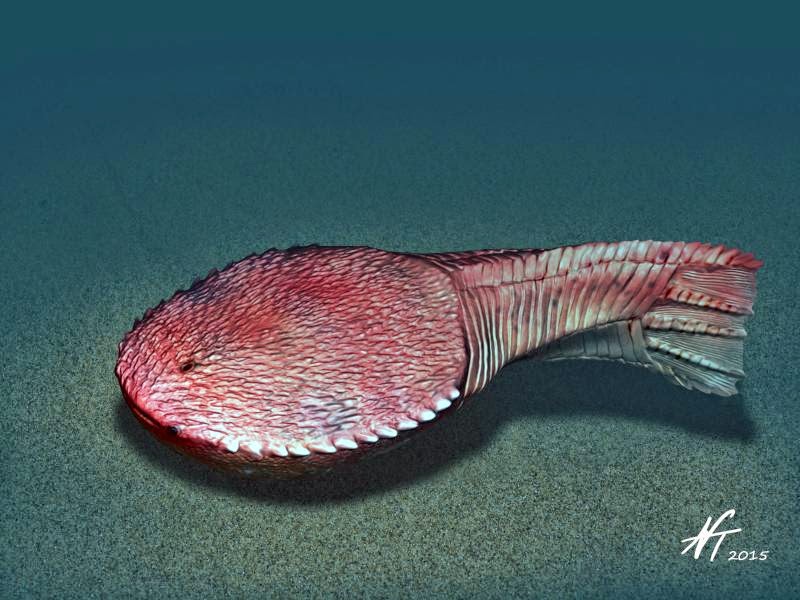
Reconstruction of the Early Devonian heterostracan fish Ctenaspis dentata by Nobu Tamura (Creative Commons Attribution 4.0 International license).
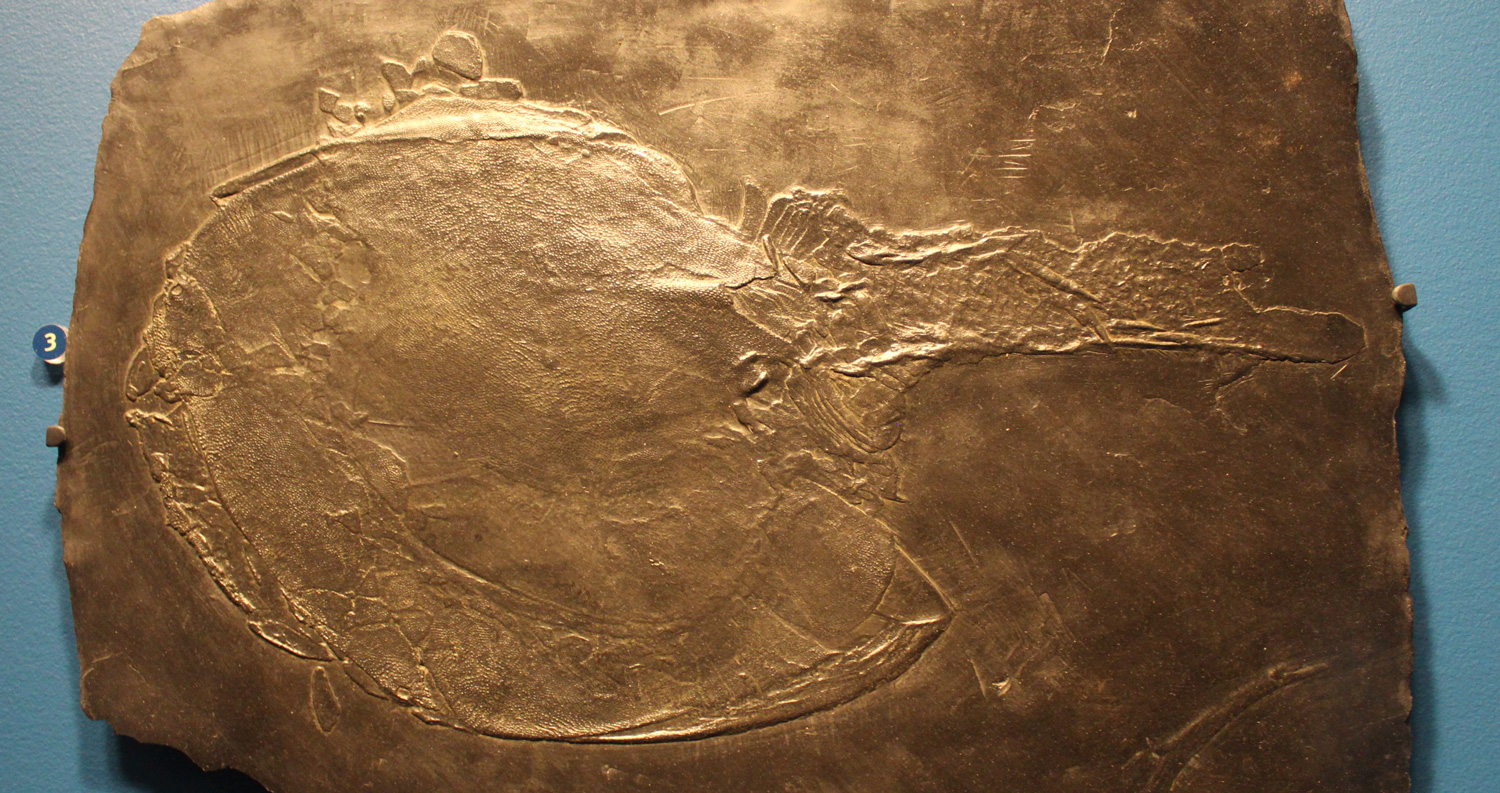
A cast of the Devonian heterostracan fish Drepanaspis sp., on display at the Field Museum of Natural History. Photograph modified from original by Jonathan Chen (Wikimedia Commons; Creative Commons Attribution-Share Alike 4.0 International license).
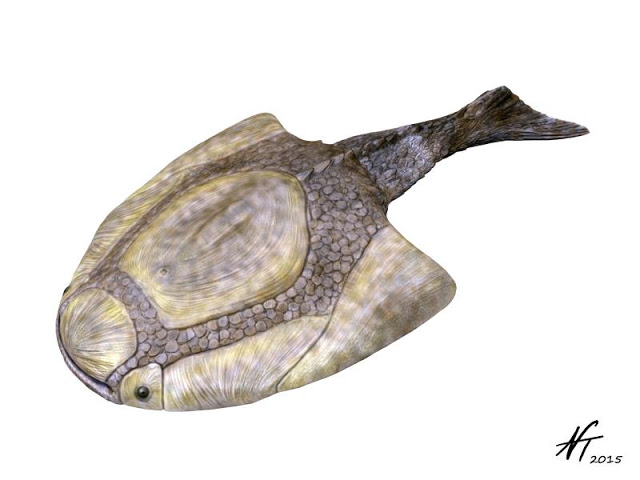
Reconstruction of the Middle Devonian heterostracan Psammolepis venyukovi by Nobu Tamura (Creative Commons Attribution-ShareAlike 4.0 International license).
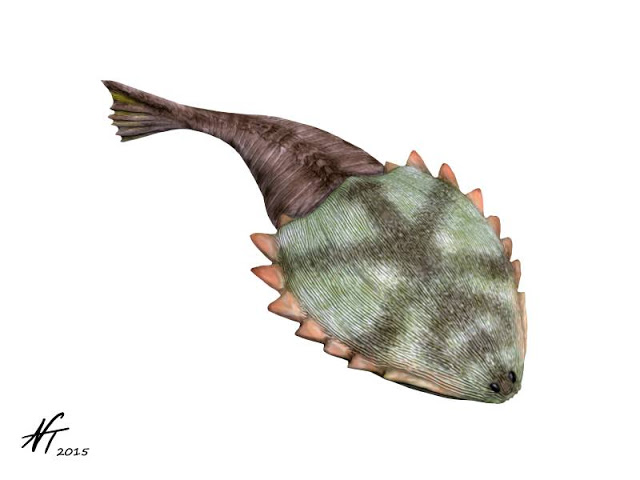
Reconstruction of the Early Devonian heterostracan Hibernaspis macrolepis by Nobu Tamura (Creative Commons Attribution-ShareAlike 4.0 International license).
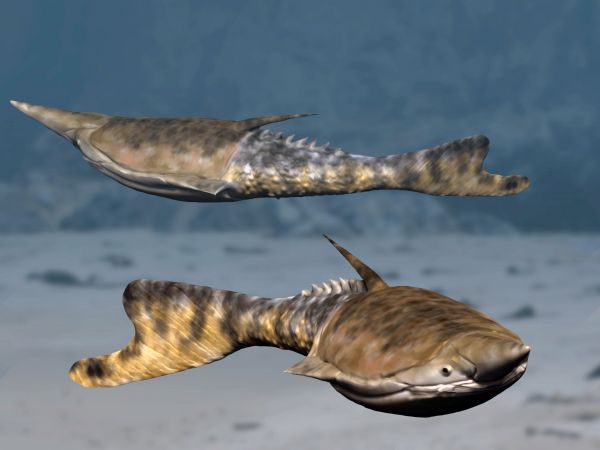
Reconstruction of the Early Devonian fish Pteraspis stensioei by Nobu Tamura (Creative Commons 3.0 Unported license).
Anaspids
Anaspid fossils are only known from the Silurian period. Their name means "without shields," owning to the fact that they lacked the large head shields that are typical of many ostracoderm groups. One feature that they all shared in common was a triangular-shaped (or, tri-radiate) spine that that projected from just behind their gill openings. Additional information about anaspids can be found on the Tree of Life website.
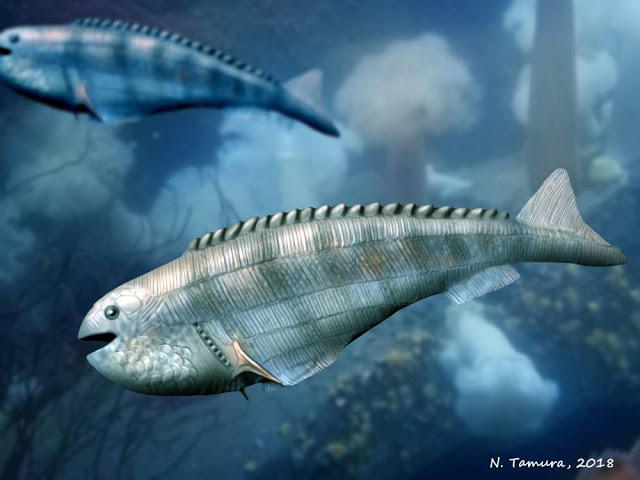
Reconstruction of the Early Devonian anaspid fish Cowielepis ritchiei by Nobu Tamura (Creative Commons Attribution-ShareAlike 4.0 International license).
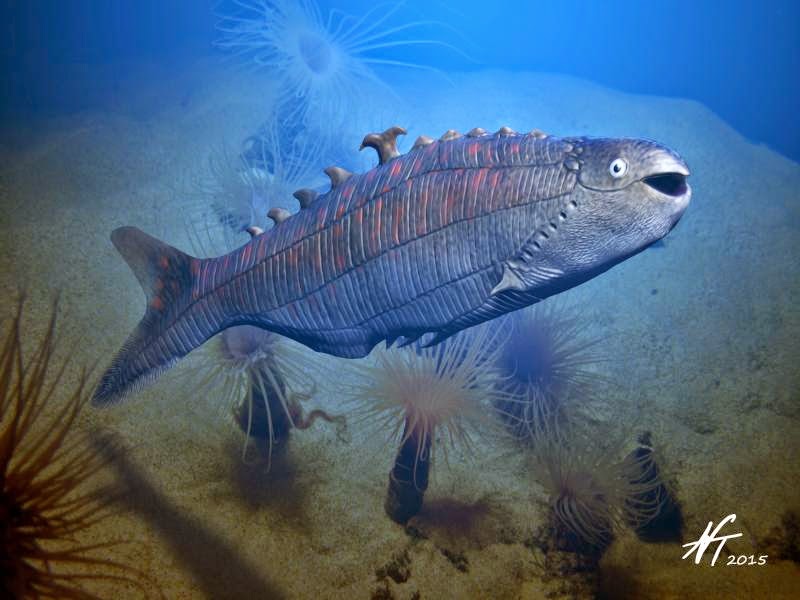
Reconstruction of the Late Silurian anaspid fish Pharyngolepis oblongus by Nobu Tamura (Creative Commons Attribution-Share Alike 4.0 International license).
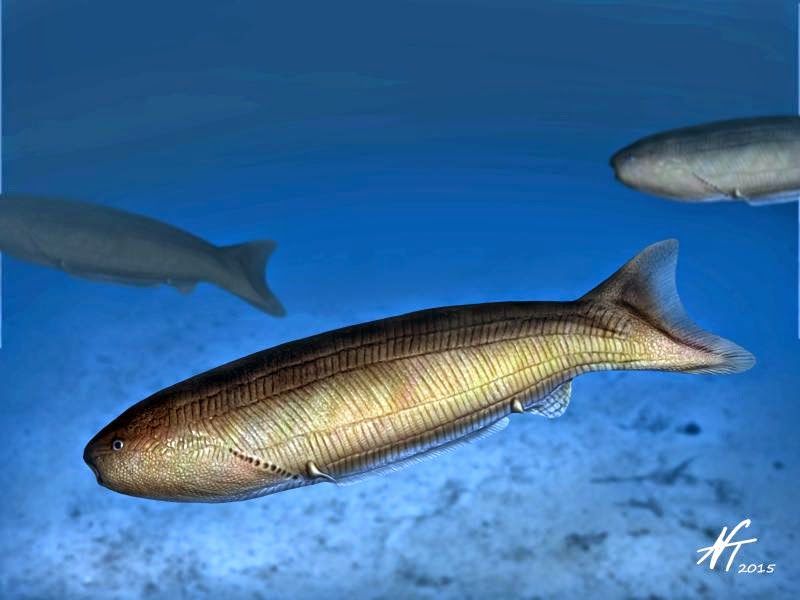
Reconstruction of the anaspid fish Birkenia elegans by Nobu Tamura (Creative Commons Attribution-Share Alike 4.0 International license).
Thelodonts
Thelodonts are not a particularly well-known group of jawless vertebrates. Their fossils are from the Silurian and Devonian periods. One feature that they seem to share in common are bodies covered by small, tooth-shaped scales that look much like those of sharks.
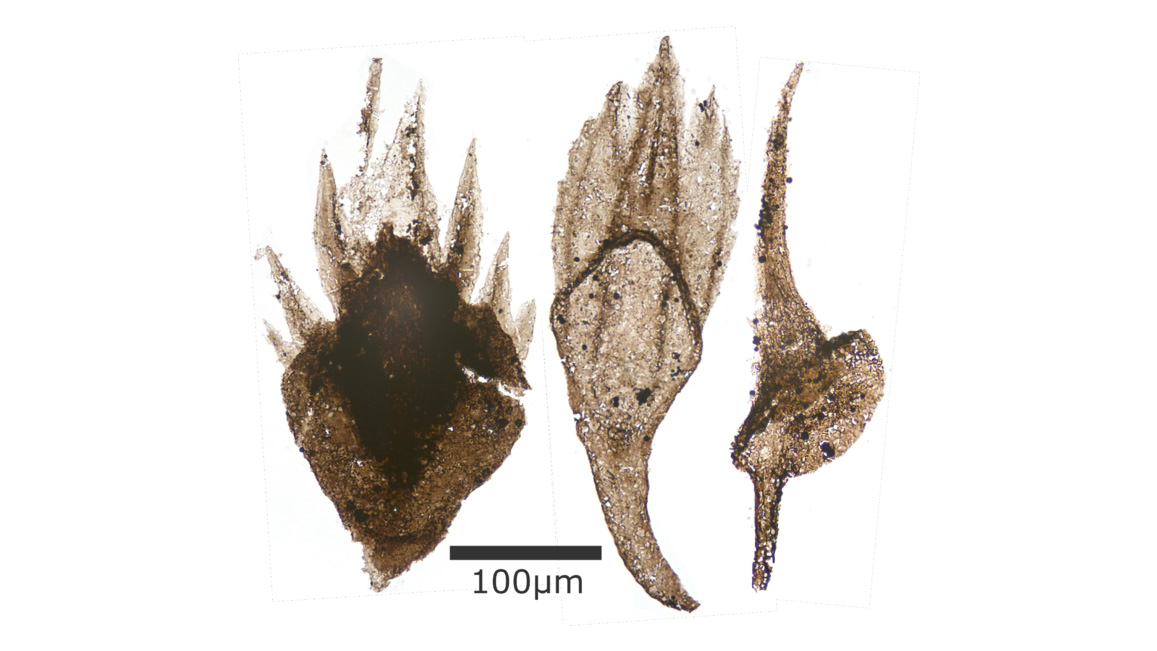
Examples of thelodont scales. Image is by "Verisimilus" (Wikimedia Commons; Creative Commons Attribution 3.0 Unported license).
The lovely reconstructions of thelodonts shown below suggest that some had bright and elaborate coloration patterns, but there is not any direct evidence that this was the case. Learn more about thelodonts on the Tree of Life website.
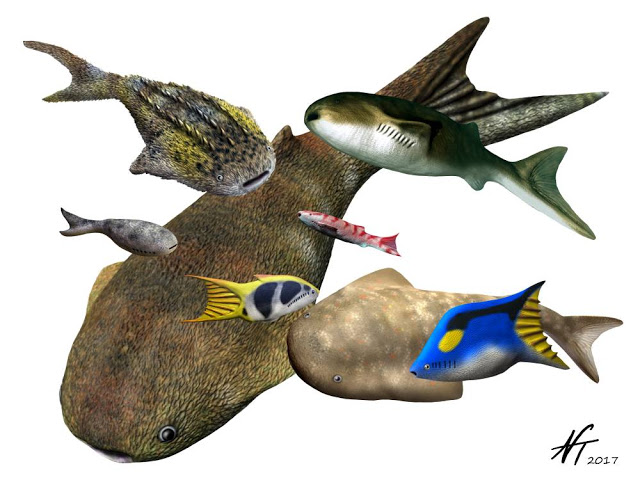
Examples of different types of thelodonts. Reconstructions by Nobu Tamura (Creative Commons Attribution-Share Alike 4.0 International license).
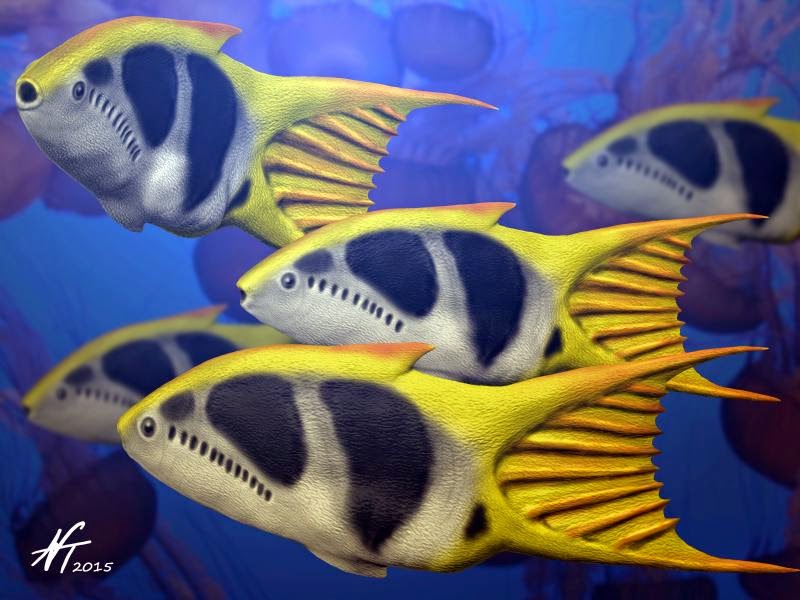
Reconstruction of the Early Devonian thelodont fish Furcacauda heintzae by Nobu Tamura (Creative Commons Attribution-ShareAlike 4.0 International license).
Galeaspids
Many galeaspids had horseshoe-shaped head shields that featured a large hole that was connected to the oral cavity (it was not the mouth, however). They lacked paired fins along the sides of their bodies. Their flattened bodies suggest that they spent most of their time on the seafloor. They had more gills (up to 45!) than any other vertebrate that ever lived. These aganthans lived during the Silurian and Devonian periods, but their fossils are only known from Vietnam and China. Learn more about Galeaspids on the Tree of Life website.
Osteostracans
Osteostracans are important because they are thought to be the group of jawless vertebrates that are most closely related to jawed vertebrates (gnathostomes). They are characterized by having large, flattened head shields and most had paired pectoral fins. Notably, portions of their internal skeleton were comprised of ossified endoskeleton (i.e., bone), which is lacking in other jawless vertebrates (which had dermal skeletons, but internal skeletons comprised of cartilage). It is likely that they spent most of their time in the benthos; specimens have been found in rocks associated with both near-shore marine and freshwater habitats. Osteostracans lived during the Silurian and Devonian periods. Around 200 species are known, all of which are from North America or Europe. Learn more about osteostracans on the Tree of Life website.
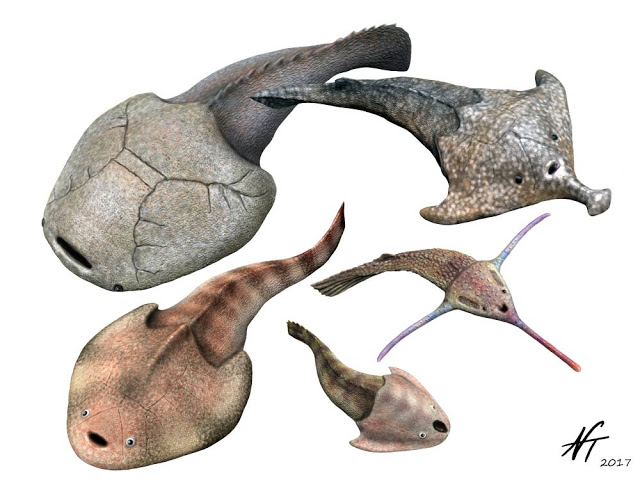
Examples of different types of galeaspids. Reconstructions by Nobu Tamura (Creative Commons Attribution-Share Alike 4.0 International license).
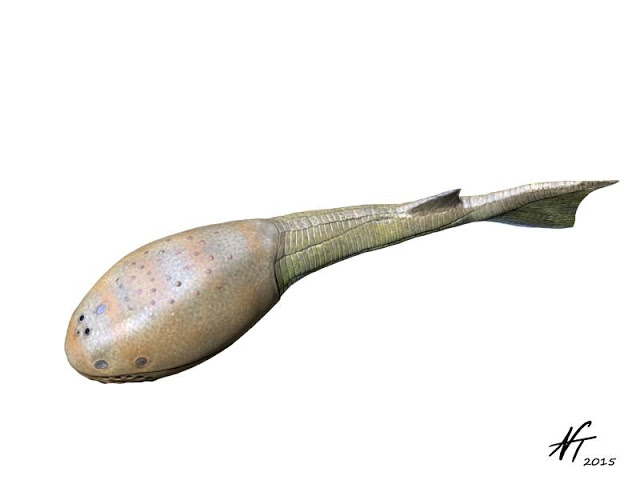
Reconstruction of the Late Silurian osteostracan fish Tremataspis mammillata by Nobu Tamura (Creative Commons Attribution-ShareAlike 4.0 International license).
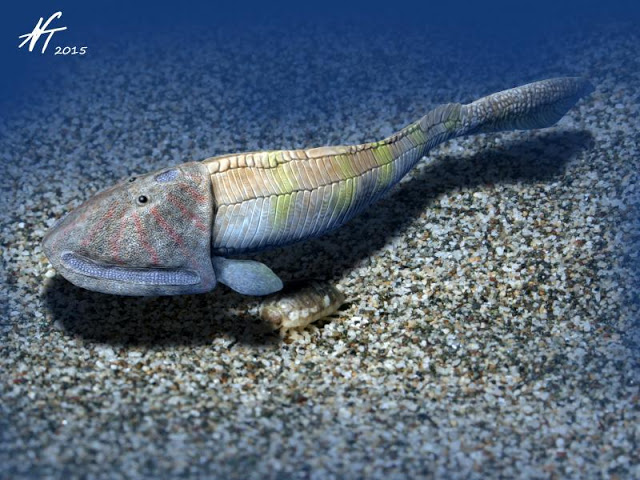
Reconstruction of the Late Silurian osteostracan Ateleaspis tesselata by Nobu Tamura (Creative Commons Attribution-ShareAlike 4.0 International license).
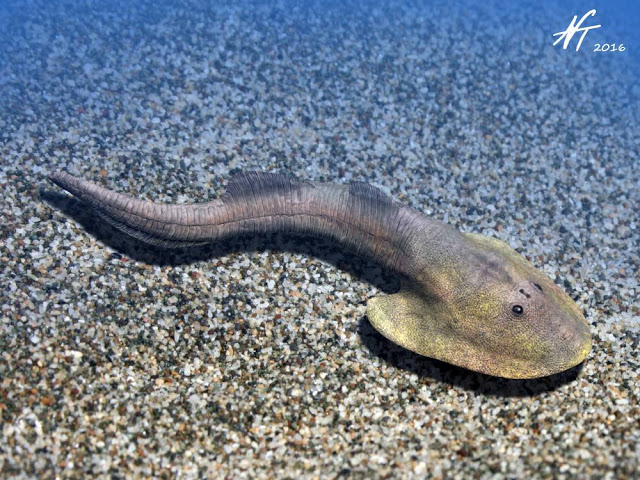
Reconstruction of the Early Devonian osteostracan Hemicyclaspis murchisoni by Nobu Tamura (Creative Commons Attribution-ShareAlike 4.0 International license).
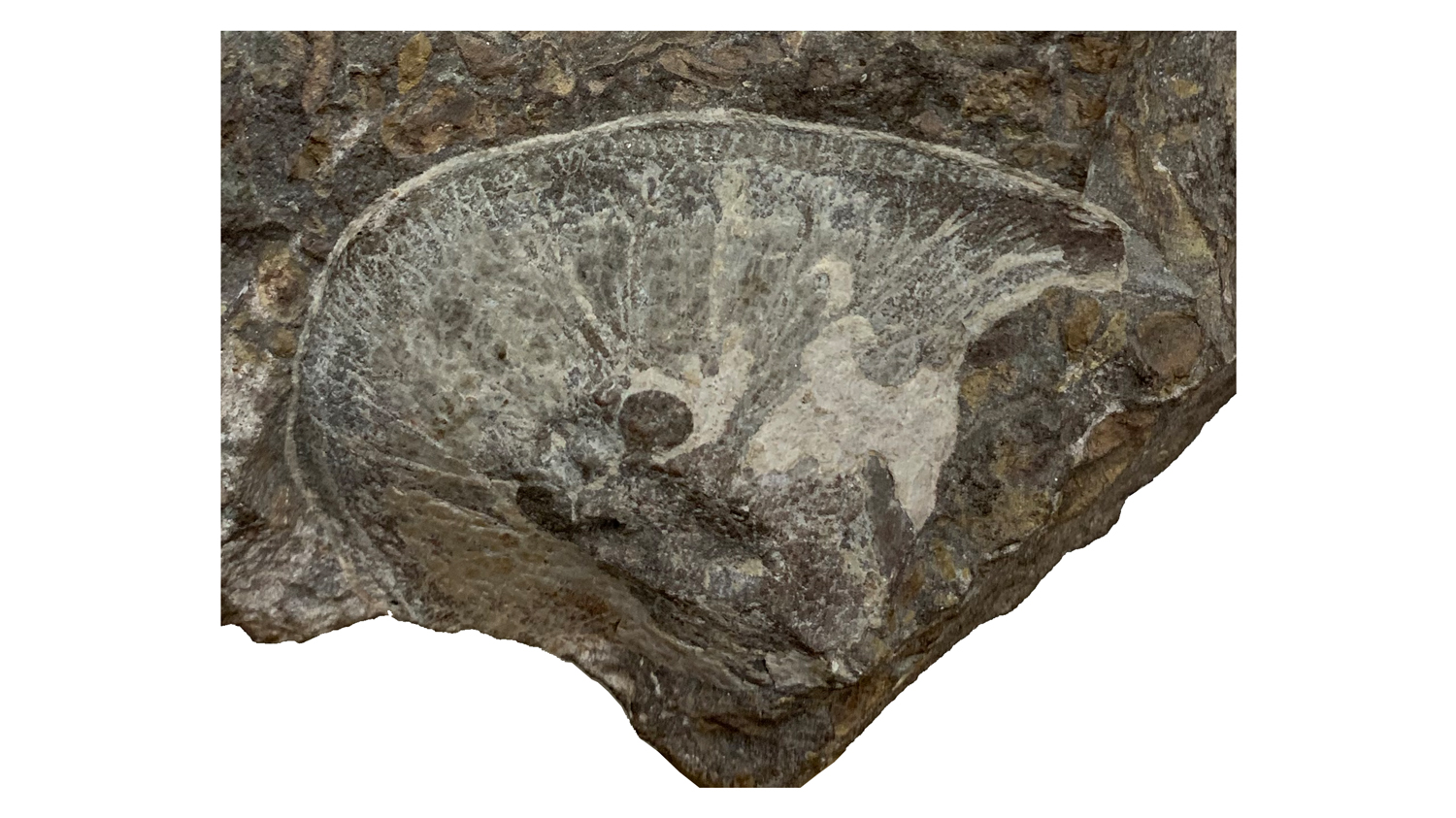
Headshield of Cephalaspis sp. from the Devonian Old Red Sandstone of England. Specimen is in the collections of the Paleontological Research Institution, Ithaca, New York.
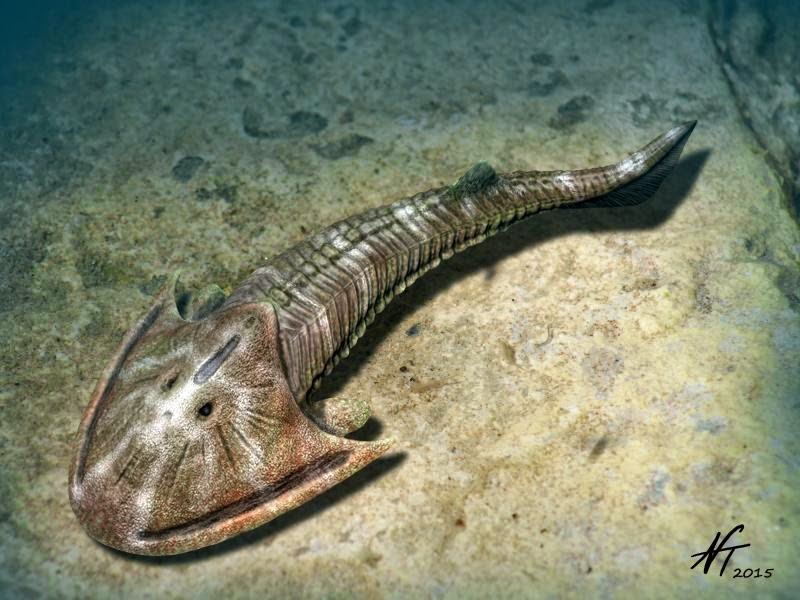
Reconstruction of the Early Devonian osteostracan fish Cephalaspis lyelli by Nobu Tamura (Creative Commons Attribution-ShareAlike 4.0 International license).
Summary
With the exception of the cyclostomes (hagfish and lampreys), all jawless vertebrates are now extinct, including the eel-like conodonts and "ostracoderms." The next major innovation in vertebrate evolution was the development of jaws, which marked the origin of the gnathostomes. This transition, as well as examples of some of the ostracoderms described above, are reviewed in the video below.
"When Fish Wore Armor" by PBS Eons (YouTube).
References and Further Reading
Bardack, D., and E. S. Richardson, Jr. 1977. New agnathous fishes from the Pennsylvanian of Illinois. Fieldiana Geology v. 33, no. 26.
Brazeau, M. D., and V. de Winter. 2015. The hyoid arch and braincase anatomy of Acanthodes support chondrichthyan affinity of 'acanthodians'. Proceedings of the Royal Society B 282: 20152210.
Brazeau, M. D., and M. Friedman. 2015. The origin and early phylogenetic history of jawed vertebrates. Nature 520: 490-497.
Briggs, D. E. G., E. N. K. Clarkson, and R. J. Aldridge. 1983. The conodont animal. Lethaia 16: 1-14.
Chang, M., F. Wu, D. Miao, and J. Zhang. 2014. Discovery of fossil lamprey larva from the Lower Cretaceous reveals it three-phased life cycle. Proceedings of the National Academy of Sciences of the United States of America 111(43): 15486-15490.
Donoghue, P. C. J. 2017. Evolution: Divining the nature of the ancestral vertebrate. Current Biology 27: R259-281.
Donoghue, P. C. J., and J. N. Keating. 2014. Early vertebrate evolution. Palaeontology 57(5): 879-893.
Epstein, A. G., J. B. Epstein, and L. D. Harris. 1977. Conodont color alteration - An index to organic metamorphism. United States Geological Survey Professional Paper 995: 27 pp.
Friedman, M., and L. C. Sallan. 2012. Five hundred million years of extinction and recovery: a phanerozoic survey of large-scale diversity patterns in fishes. Palaeontology 55(4): 707-742.
Gess, R. W., M. I. Coates, and B. S. Rubidge. 2006. A lamprey from the Devonian period of South Africa. Nature 443: 981-984.
Goudemand, N., M. J. Orchard, S. Urdy, H. Bucher, and P. Tafforeau. 2011. Synchrotron-aided reconstruction of the conodont feeding apparatus and implications for the mouth of the first vertebrates. Proceedings of the National Academy of Sciences of the United States of America 108(21): 8720-8724.
Heimberg, A. M., R. Cowper-Sallari, M. Sémon, P. C. J. Donoghue, and K. J. Peterson. 2010. microRNAs reveal the interrelationships of hagfish, lampreys, and gnathostomes and the nature of the ancestral vertebrates. Proceedings of the National Academy of Sciences of the United States of America 107(45): 19379-19383.
Janvier, P. 2015. Facts and fancies about early fossil chordates and vertebrates. Nature 520: 483-489.
Janvier, P., and R. S. Sansom. 2016. Fossil hagfish, fossil cyclostomes, and the lost world of "ostracoderms". Pp. 73-93 in: Edwards, S. L., and G. G. Goss, Hagfish Biology, CRC Press.
Keating, J. N., C. L. Marquart, F. Marone, and P. C. J. Donoghue. 2018. The nature of aspidin and the evolutionary origin of bone. Nature Ecology & Evolution 2: 1501-1506.
Miyashita, T., M. I. Coates, R. Farrar, P. Larson, P. L. Manning, R. A. Wogelius, N. P. Edwards, J. Anné, U. Bergmann, A. R. Palmer, and P. J. Currie. 2019. Hagfish from the Cretaceous Tethys Sea and a reconciliation of the morphological-molecular conflict in early vertebrate phylogeny. Proceedings of the National Academy of Sciences of the United States of America 116(6): 2146-2151.
Sansom, R. S., E. Randle, and P. C. J. Donoghue. 2015. Discriminating signal from noise in the fossil record of early vertebrates reveals cryptic evolutionary history. Proceedings of the Royal Society B 282: 20142245.
Sweet, W. C., and P. C. J. Donoghue. 2001. Conodonts: Past, present, future. Journal of Paleontology 75(6): 1174-1184.
Waid, C. B. T., and B. D. Cramer. 2017. Telychian (Llandovery, Silurian) conodonts from the LaPorte City Formation of eastern Iowa, USA (East-Central Iowa Basin) and their implications for global Telychian conodont biostratigraphic correlation. Palaeontologia Electronica 20.2.39A.
Yong, E. 2019. No one is prepared for hagfish slime. The Atlantic, January 23, 2019.
Content usage
Usage of text and images created for DEAL: Text on this page was written by Jonathan R. Hendricks. Original written content created by Jonathan R. Hendricks for the Digital Encyclopedia of Ancient Life that appears on this page is licensed under a Creative Commons Attribution-NonCommercial-ShareAlike 4.0 International License. Original images created by Jonathan R. Hendricks are also licensed under Creative Commons Attribution-NonCommercial-ShareAlike 4.0 International License.
Content sourced from other websites: Attribution, source webpage, and licensing information or terms of use are indicated for images sourced from other websites in the figure caption below the relevant image. See original sources for further details. Attribution and source webpage are indicated for embedded videos. See original sources for terms of use. Reproduction of an image or video on this page does not imply endorsement by the author, creator, source website, publisher, and/or copyright holder.