Land plants occur in a clade with a few groups of algae. Algae can be defined as eukaryotic, photosynthetic (autotrophic) organisms that are not land plants. Algae include such organisms as dinoflagellates (some of which cause red tides), kelps, diatoms, Euglenia, and other organisms. The algal groups that form a clade with the land plants include the glaucophytes, the red algae, and the green algae. All members of the clade including land plants and their algal relatives share the synapomorphy of having a primary plastid. This plastid is descended from cyanobacteria that lived in an endosymbiotic relationship with their common ancestor. Over generations, the cyanobacteria lost the ability to live on their own and became wholly incorporated into the plant cell as organelles. Plastids play a number of roles in the plant cell. Green plastids, or chloroplasts, are the cellular structures involved in photosynthesis.
The land plants are most closely related to the green algae, which together form a clade called Viridiplantae ("green plants"). Members of Viridiplantae can be grouped in part by their photosynthetic pigments (presence of chlorophyll b, absence of phycobilins). Viridiplantae is itself divided into two sister groups: chlorophytes and streptophytes. Streptophytes include the land plants and their closest green algal relatives.
The green algal relatives of land plants
The green algae most closely related to the land plants are freshwater forms in the orders Charales (stoneworts), Coleochaetales, and Zygnematales (conjugating green algae). Traditionally, Charales and Coleochaetales have been considered nearest land plants in part because these algae share some distinctive land plant features, such as oogamy (gametes are differentiated into nonmotile egg cells and motile sperm cells) and the presence of plasmodesmata (threadlike connections between the living contents of adjacent cells). Some molecular phylogenetic studies, however, suggest that Zygnematales are most closely related to land plants. Regardless, all of these green algal groups share at least one important difference from the land plants: their life cycles lack a multicellular sporophyte generation.
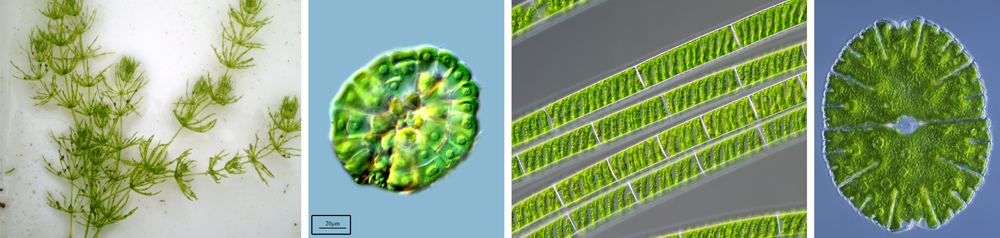
Examples of green algae from groups thought to be closely related to land plants. From left to right: Chara braunii, Charales; Coleochaete scutata, Coleochaetales; Spirogyra, Zygnematales; Micrasterias, Zygnematales. Image credits: Chara braunii (Show_ryu, Wikimedia Commons, CC BY-SA 3.0); Coleochaete scutata (Proyecto Agua, flickr, CC BY-NC-SA 2.0); Spirogyra (Wiedehopf20, Wikimedia Commons, CC BY-SA 4.0); Micrasterias (Frank Fox, Wikimedia Commons, CC BY-SA 3.0 DE). Images have been modified from originals.
The living green algae most closely related to land plants have a haplontic life cycle, also known as a zygotic life cycle (for zygotic meiosis. i.e., a life cycle in which the zygote undergoes meiosis). A haplontic life cycle can be summarized as follows: 1) Haploid organisms produces haploid gametes, or sex cells. 2) These gametes fuse in the process of fertilization to form a diploid zygote. 3) The zygote undergoes meiosis to produce haploid daughter cells. 4) The haploid daughter cells grow into the next generation of haploid organisms. Because the green algae most closely related to land plants, whether unicellular or multicellular forms, are haploid, their life cycles include only the equivalent of the gametophyte generation in land plants.
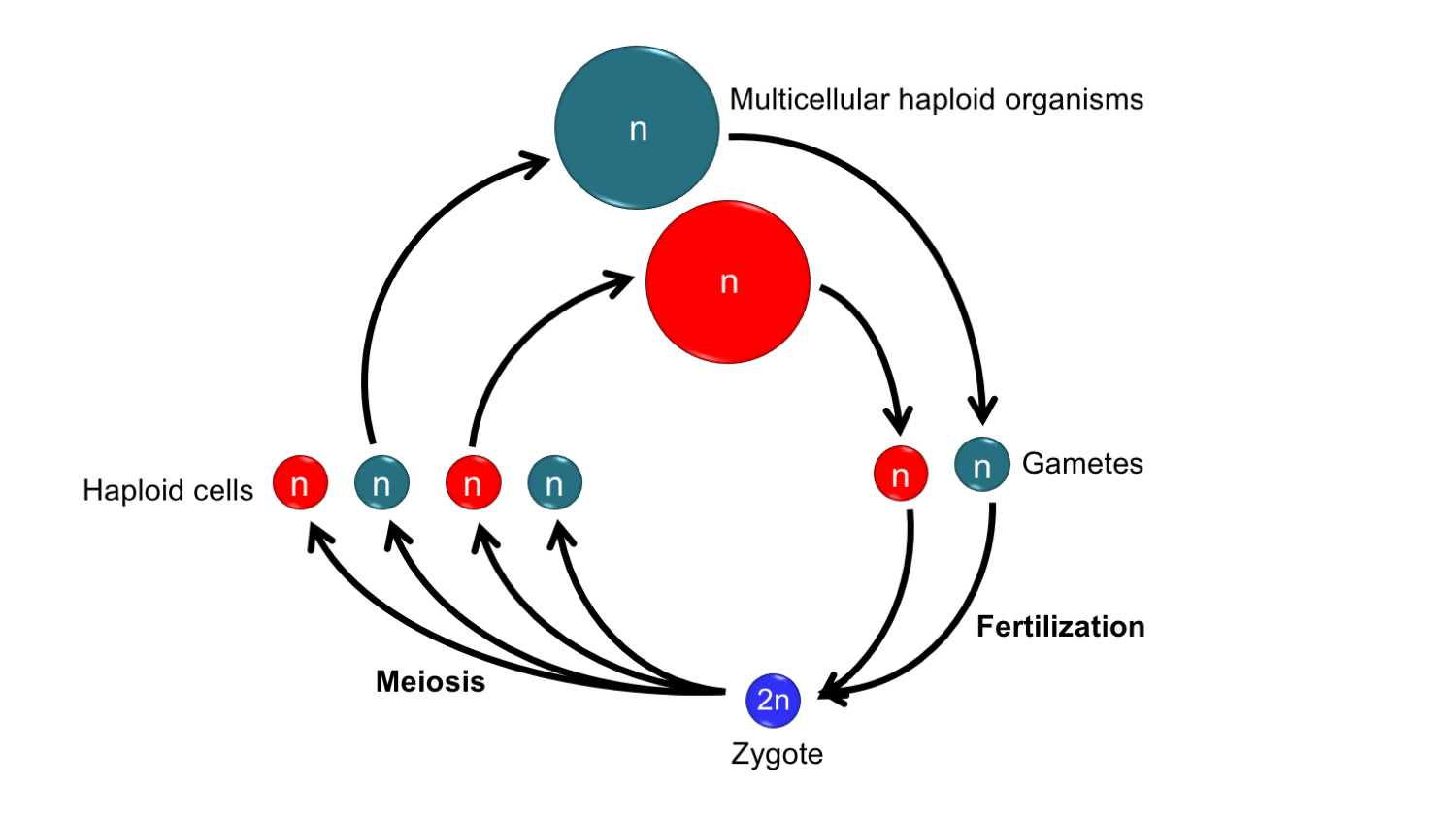
A haplontic life cycle in a multicellular organism. In this life cycle, a multicellular haploid organism gives rise to gametes (sex cells). The gametes fuse to form a zygote. The zygote undergoes meiosis to produce haploid cells. The haploid cells grow into haploid multicellular organisms to complete the life cycle.
Origin of the land plant sporophyte
The first evidence of the origin of land plant sporophytes is not in the form of macrofossils, or body fossils of sporophytes. Rather, the first evidence for the origination of land plants is the appearance of spores with resistant sporopollenin walls in the Ordovician period. Land plant spores can be identified in the early Paleozoic fossil record based on the presence of sporopollenin in the spore walls. Some of these early spores actually occur in groups of fours (tetrads), which indicates they were likely formed by meiosis. The earliest land plant spores are called cryptospores (crypto- from the Greek kryptós, hidden), and characteristically lack the Y-shaped scar (trilete mark) found on many land plant spores. The earliest cryptospores thought to have been produced by land plants are over 470 million years old and are thought to have been made by liverwort-like plants. Later in the Ordovician, trilete spores appear. Finally, sporophyte body fossils appear in the Silurian Period.
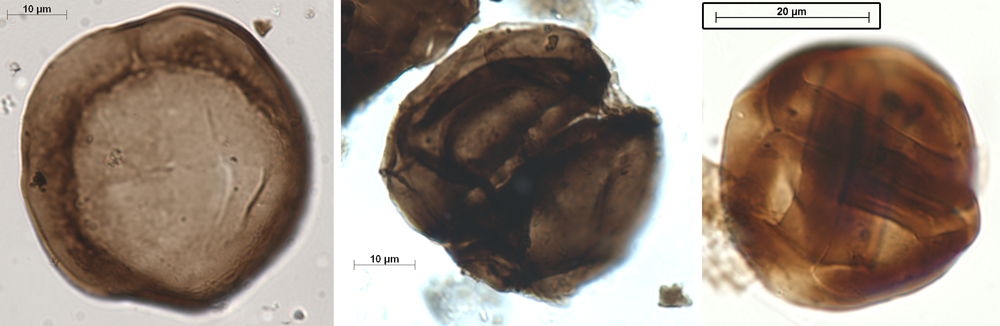
Ordovician cryptospores. Left: Cryptospore (Gneudnaspora divellomedia). Center: Cryptospores in a dyad, or group of two (Segestrespora laevigata). Right: Cryptospores in a tetrad, or group of four (Tetrahedraletes medinensis). Image credits: Gneudnaspora divellomedia, Segestrespora laevigata, and Tetrahedraletes medinensis by PhilSteem (Wikimedia Commons, CC BY-SA 4.0). Images modified from originals. Ordovician cryptospores figure by E.J. Hermsen for DEAL.
The Interpolation Theory
Where did the land plant sporophyte come from? One theory that explains the origin of the sporophyte as part of the land plant life cycle is known at the interpolation theory or the antithetic theory. In this theory, the origination of the sporophyte began when meiosis of the zygote was delayed in an organism that had a haplontic life cycle. The delay in meiosis allowed a multicellular diploid structure to arise following fertilization of an egg. This diploid sporophyte eventually become permanently inserted, or interpolated, as an extra step in the life cycle.
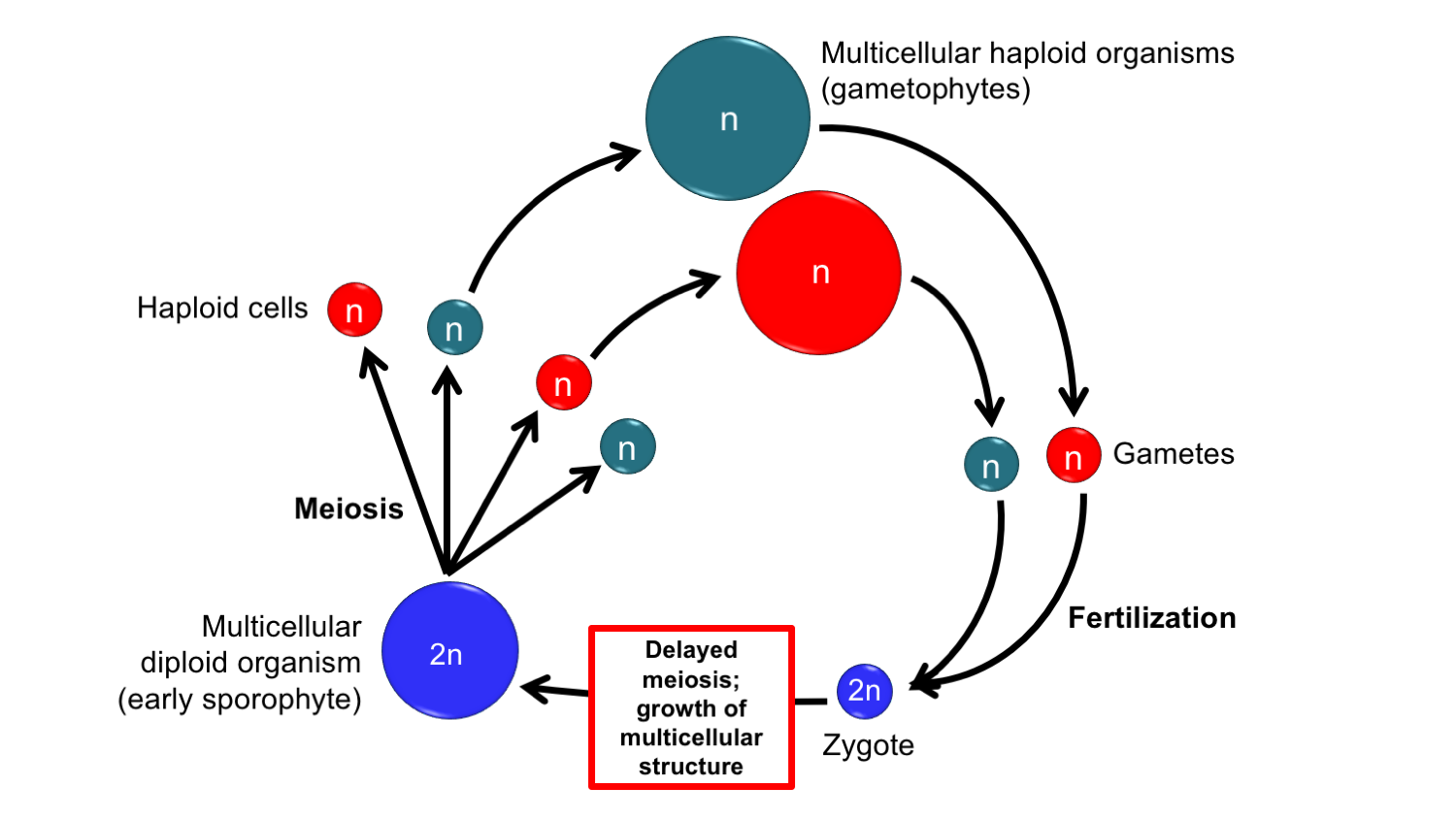
Interpolation theory. The interpolation theory posits that the sporophyte in land plants was inserted into the life cycle of an haplontic organism. The sporophyte first arose when the zygote did not immediately undergo meiosis, but instead underwent a period of grow (mitosis, or normal cell division) before meiosis occurred. The sporophyte was morphologically different from the gametophytes at the time of its origination.
Under the interpolation theory, one might make several predictions about the earliest sporophytes. First of all, the earliest sporophyte and gametophyte generations would have been heteromorphic generations (from the Greek heteros + morphē, different + form). In other words, they would likely have looked different from one another, since the sporophyte arose from the zygote by delay of meiosis. Secondly, that the earliest sporophytes might have been relatively simple in structure. Finally, that the earliest sporophytes might have developed on and been nutritionally dependent on gametophytes.
We do not have direct fossil evidence demonstrating how the first sporophytes evolved. Because the green algae most closely related to land plants lack a sporophyte generation, it is reasonable to conclude that the sporophyte is a novel innovation in the land plant lineage; in other words, that the sporophyte first arose in early land plants or their immediate ancestors.
The three basally diverging groups of living land plants are the bryophytes, or the hornworts, liverworts, and mosses. In the bryophytes, the gametophyte generation is green and persistent. The sporophyte, in contrast, is very simple and nutritionally dependent on the gametophyte. The two generations (sporophyte and gametophtye) look very different from one another. Thus, the structure and life cycle of bryophytes is consistent with the interpolation theory of the origin of the land plant sporophyte. It is unknown, however, whether the earliest multicellular sporophytes really formed directly on gametophytes, or whether the retention of the developing sporophyte evolved later than the evolution of the multicellular sporophyte itself.
The Homologous Theory
The homologous theory or transformation theory is an alternate theory for the origin of heteromorphic sporophyte and gametophyte generations in land plants. Under this theory, the green algal ancestor to land plants already had a life cycle with alternation of multicellular sporophyte and gametophyte generations. In contrast to land plants, however, these generations looked the same, or were isomorphic (from the Greek isos + morphē, equal + form). The homologous theory postulates that over time, the sporophyte and gametophyte generations diverged in structure so that they came to look different from one another in land plants. Some modern algae, like Ulva (the sea lettuce, a marine green alga) have life cycles with alternation of isomorphic generations.
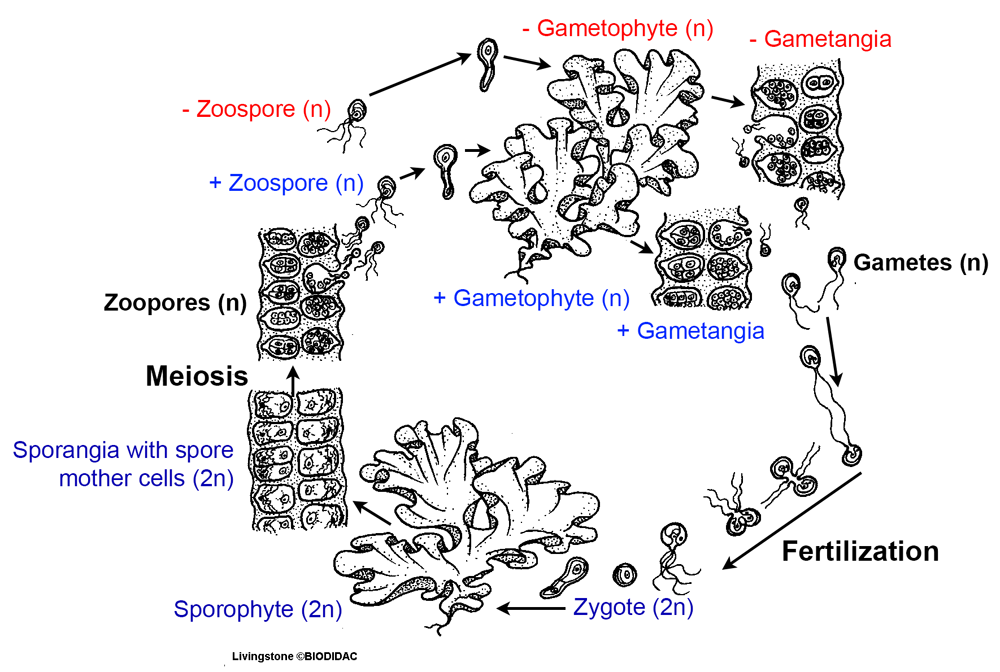
Life cycle of the sea lettuce, Ulva. Note that the steps in the life cycle of Ulva are basically the same as the steps in the land plant life cycle. Ulva is a green alga showing alternation of isomorphic generations. In other words, the sporophyte and gametophyte generations look the same in Ulva. The spores of Ulva are called zoospores (zoo- from the Greek zoion, animal) because they are motile, or able to move or swim. Image credits: Illustration of the life cycle of Ulva (Ivy Livingstone, BIODIDAC, CC BY-NC 4.0). Image modified from original.
The major distinction between the homologous theory and the interpolation theory is in the sequence of events. Under the homologous theory, alternation of generations arose first, with sporophyte and gametophyte generations diverging in form later. Under the interpolation theory, origination of heteromorphic generations happened concurrently with origination of sporophyte (and the alternation of generations) in the land plant life cycle.
The homologous theory is not supported by our current understanding of the evolutionary relationships between green algae and land plants, because the green algae that show alternation of generations in their life cycles are closely related to the land plants. The best explanation for the evidence at hand is that alternation of generations evolved independently more than once in the streptophyte clade, and that alternation of generations and heteromorphic gametophyte and sporophyte generations evolved concurrently in the early land plants or their immediate algal ancestors.
Why the sporophyte?
Elaboration of the sporophyte
The story of land plant macroevolution, especially when viewed through a paleobotanical lens, is the story of the elaboration of the sporophyte generation, with corresponding reduction in the gametophyte generation. Recall that some of the first evidence for the origination of land plants is the occurrence of cryptospores in the Ordovician. Thus far, however, no fossil evidence preserving the structure of the earliest, simplest sporophytes has been discovered, and there is a big time gap between the first land plant cryptospore records and the first sporophyte body fossils. Most of the fossil evidence documenting the structure of early land plant sporophytes comes from the Silurian to Devonian periods. The physical evidence for the structure of sporophytes can be combined with mapping of characters on phylogenetic trees and analysis of the structure and development of modern plants to provide a portrait of the evolution of the sporophyte complexity in the Ordovician to Silurian periods.
The earliest sporophytes were probably like the sporophytes of bryophytes. Thus, a sporophyte would have been a simple organism with a single-unbranched stalk bearing one sporangium at its tip. In fact, the earliest sporophytes may have been even simpler, consisting, for example, of a single, stalkless sporangium. Early sporophytes may have been anchored in the gametophyte tissue by a foot at the base of the stalk. The foot is the structure through which the sporophyte would have absorbed nutrients from the gametophyte. Early sporophytes were probably dependent on their mother gametophytes for nutrition; in other words, they would not have been able to sustain themselves independently through photosynthesis. The earliest sporophytes would have had no roots or leaves.
Stomata, or pores surrounded by guard cells, were one of the earliest sporophyte innovations to appear. Stomata allow for the exchange of gases. In many plants, the guard cells control the opening and closing of the stomata, to balance gas exchange necessary to carry on life processes (i.e., photosynthesis) against the loss of water. Stomata are found in all living plant sporophytes except the sporophytes of liverworts, which are thought to be the first-diverging group of living land plants. It is thus inferred that stomata may have appeared in the Ordovician.
Sporophytes then began to increase in complexity. In the polysporangiophytes ("many-sporangium plants"), the sporophyte stalk began to grow and branch at its tip. Eventually sporophytes came exhibit indeterminate growth. This meant that a single sporophyte could grow and elongate indefinitely from specialized growing regions (meristems) at the tips of its axes. A single sporophyte could also produce many multiple sporangia over the course of its life, and thus many more spores.
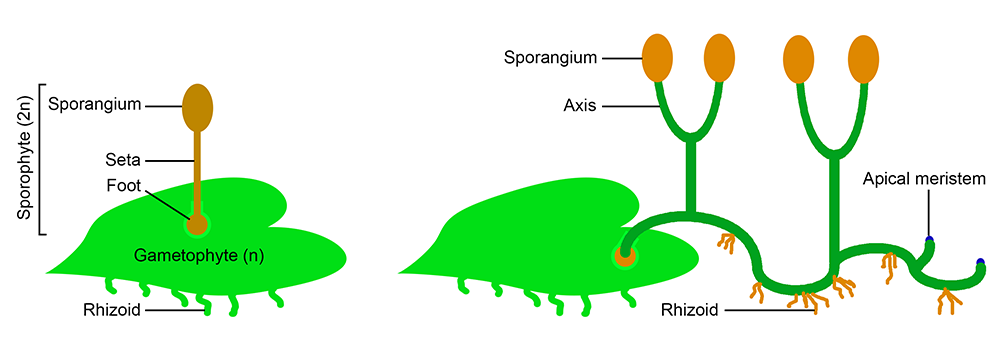
Early evolution of the sporophyte. Left: A typical bryophyte-like sporophyte, probably similar to some of the earliest land plant sporophytes. The sporophyte consists of a foot, which anchors the sporophyte in the gametophyte tissue and absorbs nutrition; a seta, or stalk; and a sporangium, or spore capsule. Some bryophyte sporophytes lack even a stalk, and consist of a foot and sporangium. Right: Sporophyte of an early polysporangiophyte. The sporophyte would have begun its life attached to and drawing nutrition from the gametophyte. However, it would have eventually have become independent. Note that the sporophyte has apical meristems, or regions where apical growth occurs. Also note that the sporophyte is green (photosynthetic) and anchored to the ground by rhizoids. Rhizoids are anchoring structures consisting of one cell or a row of cells (contrast to roots, which are more complex structures found in vascular plants). Image modified after Figure 8 in Ligrone et al. (2012).
Sporophytes also evolved specialized conducting tissues to shuttle food (phloem) and water (xylem). The origination of xylem in the fossil record is signified by the appearance of the tracheid, a type of water-conducting cell. These vascular tissues allowed tracheophytes (vascular plants) to grow taller, as they could now shuttle water from the ground to the tips of aerial axes and food amongst regions of food synthesis, storage, and growth. Both branching sporophytes and tracheids are known from Silurian fossils.
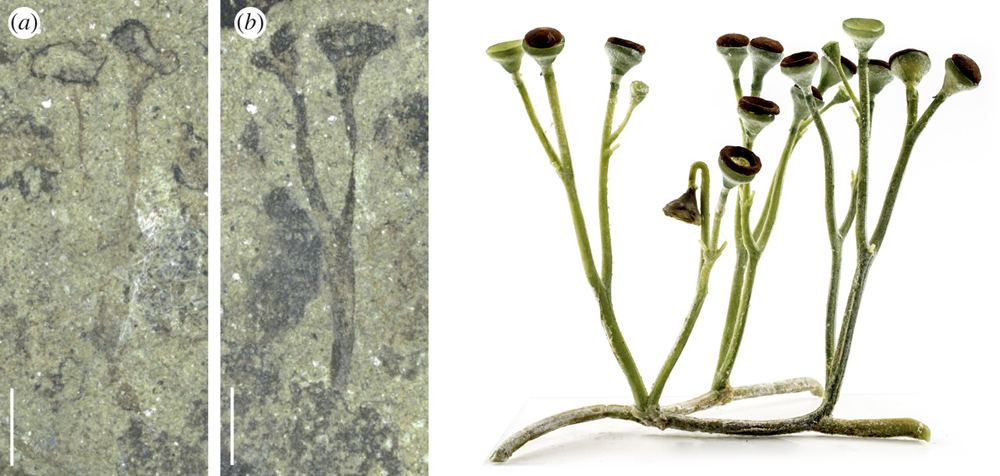
Cooksonia pertoni, an early tracheophyte (Late Silurian). Left (A-B). Branching axes of Cooksonia pertoni from the Late Silurian of the UK. Note terminal sporangia. (scale bar = 2 mm). Right. Reconstruction of a Cooksonia sporophyte. Image credits: Cooksonia pertoni fossils modified from Figure 2 in Edwards & Kendrick (2015), CC BY 4.0); Cooksonia model (MUSE-Science Museum of Trento, Wikimedia Commons, CC GY-SA 3.0).
Specialization of non-reproductive organs took place, with the evolution of leaves and roots. As the sporophyte body became larger and more elaborate, it also required more support. Wood, which is made up of xylem (water-conducting tissue), evolved. Leaves and wood appear in the fossil record by the Devonian, with roots also inferred to evolved within the Silurian to Devonian.
Reproductive structures also became more complex. Initially, individual sporangia formed at the tips of axes (stems). Later, they were borne on sporophylls, or sporangium-bearing leaves. The sporophylls then became aggregated into strobili, or cones. A flower can be considered a type of strobilus.
It should be noted that many of these structural innovations occurred more than once in land plants. For example, wood, leaves, and strobili have evolved separately in unrelated groups. Thus, the evolution of the sporophyte is not a story of simple, linear progression, but is instead a process of repetitive structural evolution and innovation.
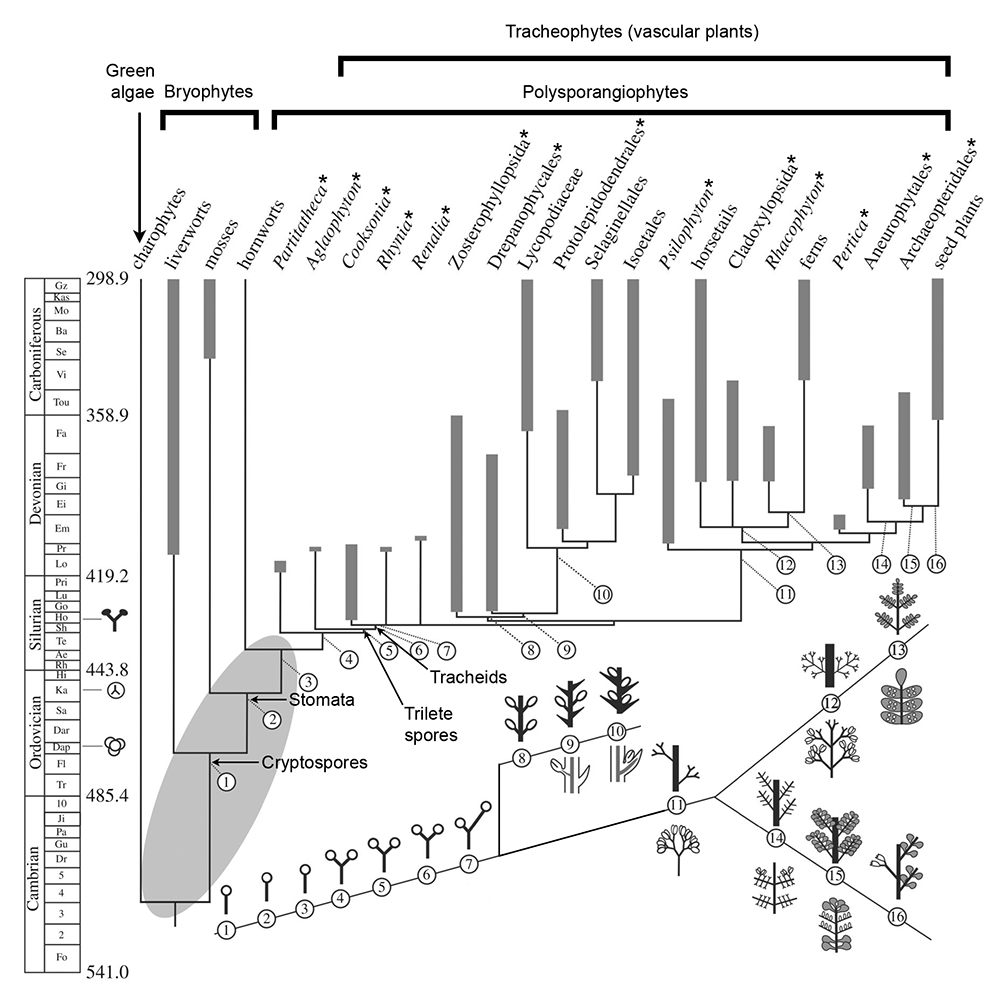
Key innovations in the structure of the land plant sporophyte during the Paleozoic. This diagram shows a hypothetical phylogeny for land plants scaled to time. Extinct taxa are marked by an asterisk (*). A portion of the Geologic Time Scale is shown on the left, with the numerical ages of boundaries between time periods marked in millions of years before present. Fossil evidence for land plants (bottom to top: cryptospores, trilete spores, and sporophytes) is marked on the time scale. Key innovations inferred to have occurred in the structure of the land plant sporophyte are shown underneath the phylogenetic tree. The number for each innovation is mapped to its position on the phylogenetic tree. 1-3. Unbranched sporophyte with terminal sporangium (spore capsule). 4-6. Sporophyte with isotomous (equal) apical branching. 7. Sporophyte with unequal apical branching. 8-10. Evolution of leaves and lateral sporangia in the lycophyte lineage. 11-16. Evolution of branching, leaves, and sporangial position in the euphyllophyte lineage (including horsetails, ferns, seed plants, and extinct relatives). Image modified from Figure 1 (CC BY 4.0) in Harrison & Morris (2017).
Selected references & additional reading
Edwards, D., and P. Kendrick. 2015. The early evolution of land plants, from fossils to genomics: a commentary on Lang (1937) 'On the plant-remains from the Downtonian of England and Wales.'Philosophical Transactions of the Royal Society B, Biological Sciences 370: 20140343. http://dx.doi.org/10.1098/rstb.2014.0343
Harrison, C.J., and J.L. Morris. 2017. The origin and early evolution of vascular plant shoot and leaves. Philosophical Transactions of the Royal Society B, Biological Sciences 373: 20160496. https://dx.doi.org/10.1098/rstb.2016.0496
Ligrone, R., J.G. Duckett, and K.S. Renzaglia. 2012. Major transitions in the evolution of early land plants: a bryological perspective. Annals of Botany 109: 851-871. https://doi.org/10.1093/aob/mcs017
Rubinstein, C.V., P. Gerrienne, G.S. de la Puente, R.A. Astini, and P. Steemans. 2010. Early Middle Ordovician evidence for land plants in Argentina (eastern Gondwana). New Phytologist 188: 365-369. https://doi.org/10.1111/j.1469-8137.2010.03433.x