Section contents:
- Origin of land plants
- The land plant life cycle ←
- Greek and Latin in botanical terminology
Feature image. Diagram showing the life cycle of a homosporous, free-sporing land plant. For further explanation, read below. Credit: E.J. Hermsen (DEAL).
Topics covered on this page:
Introduction
One of the defining features of land plants is their life cycle. The land plant life cycle is known as a sporic (for sporic meiosis), dibiontic, or haplodiplontic life cycle. This type of life cycle exhibits alternation of generations. In other words, to complete a full circuit of its life cycle, a land plant must produce two different types of multicellular organisms. These organisms, or generations, are called the sporophyte (spore-producing plant) and the gametophyte (gamete-producing plant). A spore is a cell that can grow into a new organism without combining with another cell. In contrast, gametes (sex cells) are cells that fuse during fertilization. In plants, the gametes are egg and sperm.
The suffix "-phyte" is from the Greek word phyton, meaning "plant." Remember, the sporophyte is the plant that makes spores, whereas the gametophyte is the plant that makes gametes.
The alternation of generations in land plants
In order to understand the concept of alternation of generations, let us examine the life cycle of a fern. Ferns have both sporophytes and gametophytes that are capable of living independently; in other words, they can sustain themselves as free-living organisms. Thus, it is easier to see how the alternation of generations works in a fern than in some other groups of land plants, like bryophytes and seed plants, where the generations remain physically connected to one another.
In a fern, the leafy plant with fronds that you may grow in your house or garden is a sporophyte. This plant is diploid, or has two sets of chromosomes in its cells (just like you do).
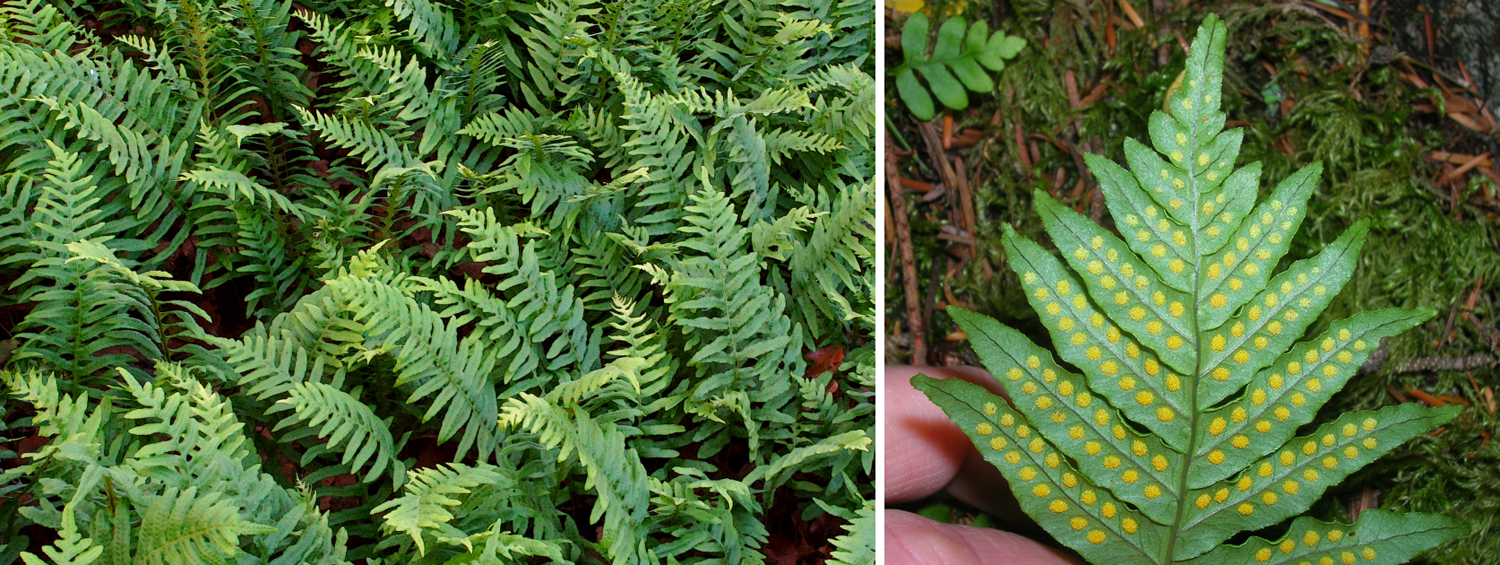
Sporophytes. Left: Licorice fern (Polypodium glycyrrhiza) fronds. Right: Underside of a licorice fern frond showing sori, or groups of sporangia (spore-producing capsules). Credits: Polypodium glycyrrhiza (John Rusk, via Wikimedia Commons, CC BY 2.0); Polypodium glycyrrhiza sori (brewbooks, via Wikimedia Commons, CC BY-SA 2.0). Images modified from originals.
Capsules develop on the undersides or edges of the fronds (leaves) of the fern sporophyte. These capsules are called sporangia. Within the sporangia, spore mother cells divide to produce spores. The spores are produced by a special type of cell division called meiosis. During meiosis, a single diploid parent cell divides to give rise to four haploid daughter cells. A haploid cell has only one set of chromosomes. In the fern sporangium, the diploid parent cells are the spore mother cells and their haploid daughter cells are the spores. Thus, each spore has only one set of chromosomes. Each spore develops a tough spore wall made out of a substance known as sporopollenin.
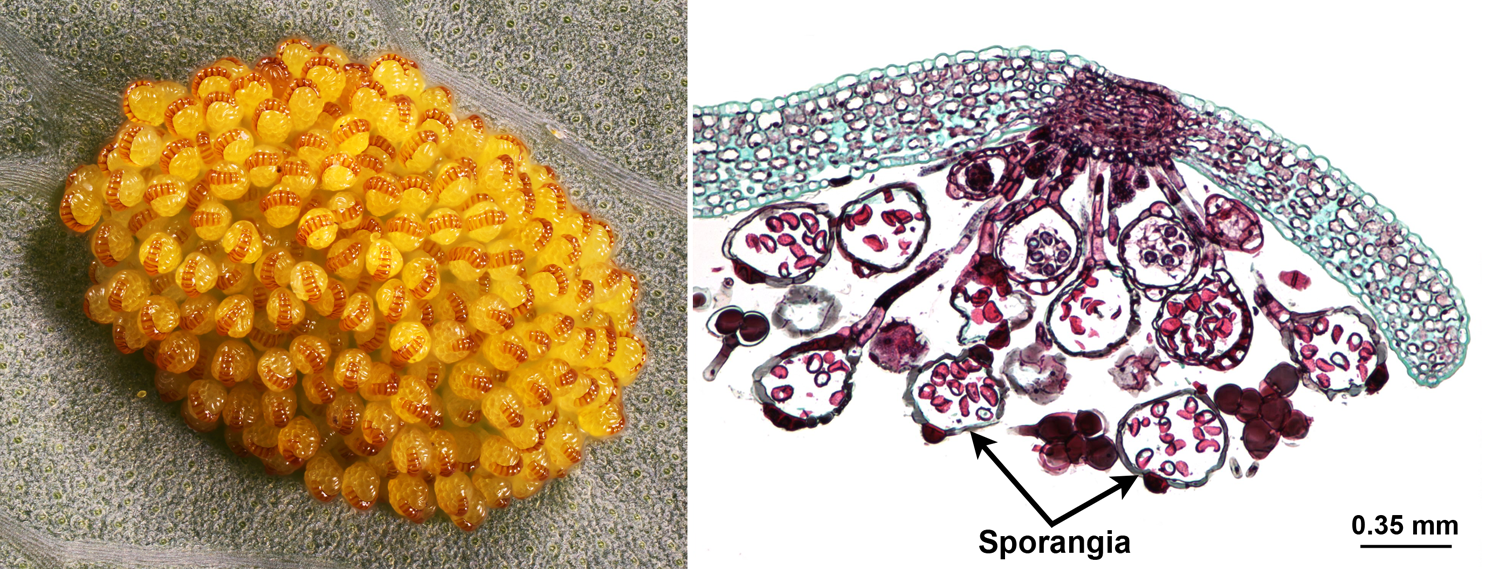
Sporangia (spore-producing capsules). Left: Golden polypody (Phlebodium aureum), underside of frond (fern leaf) with a cluster of sporangia (spore-producing capules) that have not yet opened. Right: Section through a of group of sporangia on the underside of a polypody (Polypodium) frond. Note the spores (stained dark pink) inside each sporangium. Credits: Phlebodium aureum sorus (Anatoly Mikhaltsov, via Wikimedia Commons, CC BY-SA 4.0); Polypodium sorus (Jon Houseman & Matthew Ford, via Wikimedia Commons, CC BY-SA 3.0). Images modified from originals.
The sporangia open to release the spores. If a spore lands in a favorable environment, it may germinate and grow into another type of plant, the gametophyte. In many ferns, the gametophyte is very small, thin, green, and heart-shaped. Each of the cells in a gametophyte is haploid.
Gametangia form on the gametophyte. Gametangia are structures that produce haploid gametes, or sex cells. In plants, the gametes are eggs and sperm. The type of gametangium that produces the egg is called an archegonium, whereas the type of gametangium that produces the sperm is called an antheridium. While an antheridium may produce many sperm cells, each archegonium contains only one egg cell.
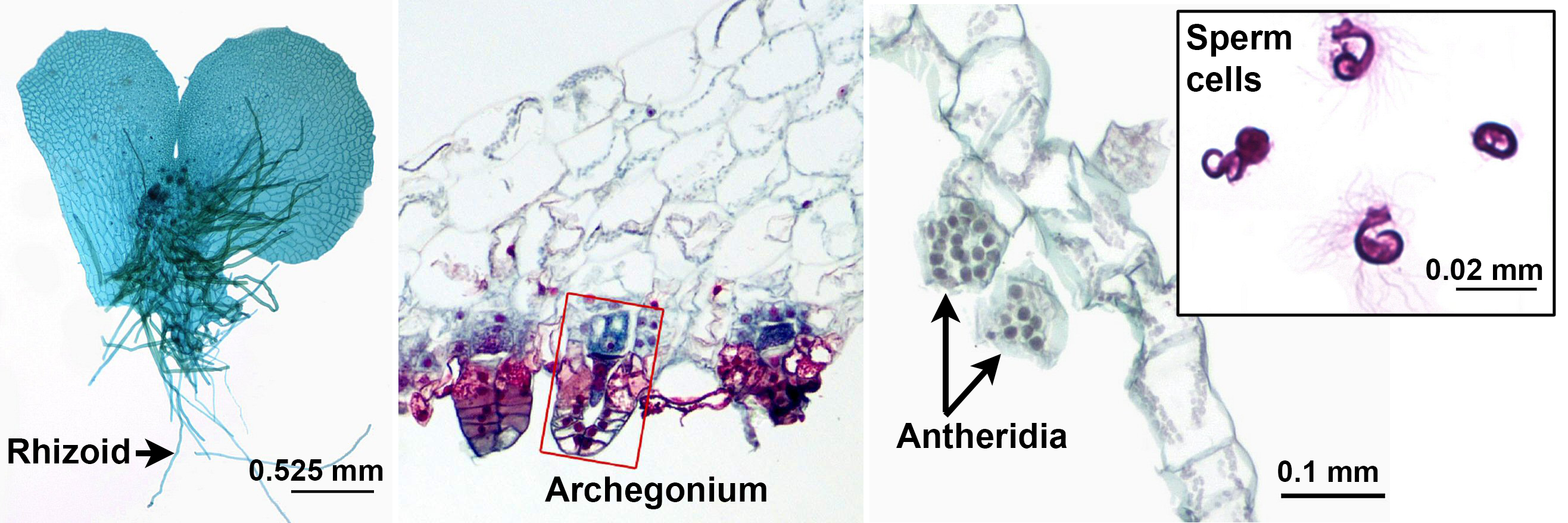
Gametophytes, gametangia, and gametes. Left: Underside of fern gametophyte showing rhizoids (anchoring structures). The gametangia develop near the notch in the gametophyte and amidst the rhizoids. Center: Archegonia, or egg-producing structures; one egg cell forms at the base of each archegonium. Right: Antheridia (sperm-producing structures) open to release the swimming sperm cells (inset, far right). Credits: Fern gametophyte, fern antheridia, and fern sperm (Jon Houseman & Matthew Ford, via Wikimedia Commons, CC BY-SA 4.0); Pteridophyta Woodwardia archegonium 01 (Bruce Kirchoff, via flickr, CC BY 2.0). Images modified from originals.
Once the sperm cells mature, the antheridia open to release the sperm. Ferns and many other groups of plants have motile sperm that swim by means of whip-like or thread-like structures called flagella. Thus, the sperm require the presence of a thin layer of water to reach an egg. A sperm cell swims through a short canal in the neck of an archegonium to reach the egg cell at the bottom.
Video showing swimming fern sperm. This video provides an explanation of the fern life cycle and shows images of swimming sperm cells. Note: This video has no sound. (Credit: Swimming fern sperm, WilliamCapman, via YouTube).
When sperm and egg fuse, a cell called a zygote is formed. This process is known as fertilization. The zygote has two sets of chromosomes, one from the sperm and one from the egg. Thus, the zygote is diploid. The zygote is the first cell of the next generation of sporophyte. The zygote begins to divide to produce a multicellular sporophyte embryo. The embryo is an immature sporophyte that is nourished (fed) by the gametophyte. Eventually, as the sporophyte grows, it will be able to sustain itself. When the sporophyte is mature, the fern life cycle is complete.
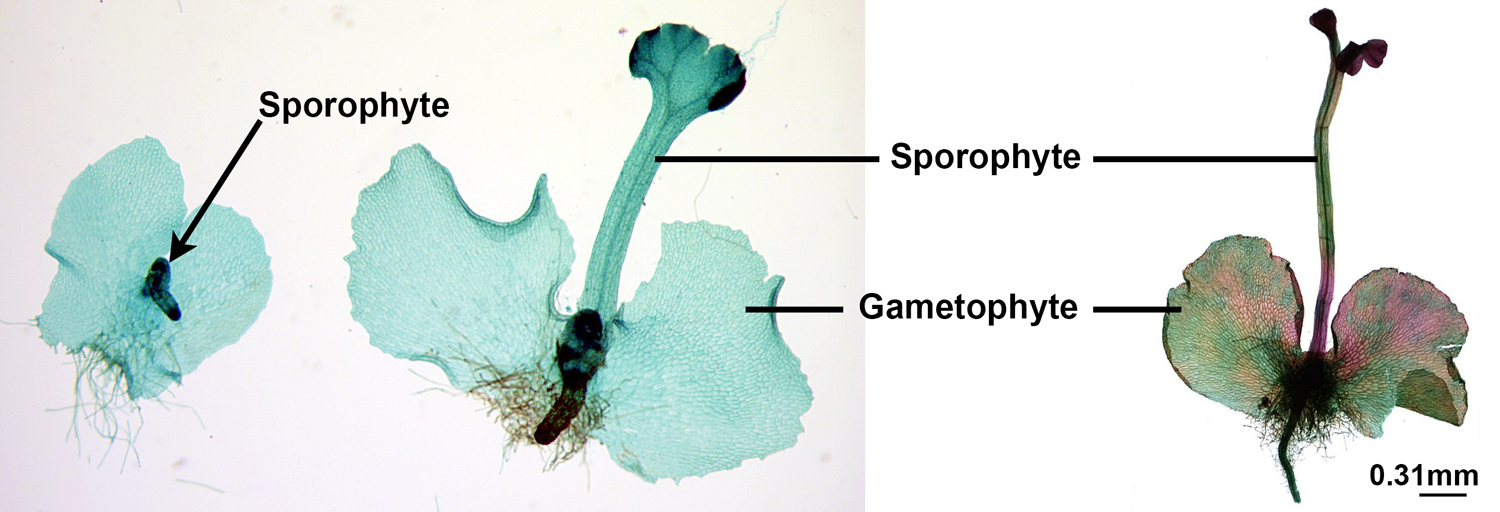
Developing sporophytes. The young fern sporophyte begins development in the archegonium of the gametophyte. As it grows, it will become an independent plant capable of sustaining itself. The gametophyte will eventually die. Credits: Whole-mount slide of sporophyte on gametophyte (Curtis Clark, via Wikimedia Commons, CC BY-SA 3.0); young sporophyte on gametophyte (Jon Houseman & Matthew Ford, via Wikimedia Commons, CC BY-SA 4.0). Images modified from originals.
While the details differ, the life cycles of all plants follow the basic steps outlined above:
- The sporophyte produces spores by meiosis.
- The spores grow into gametophytes.
- The gametophytes produce gametes (eggs and sperm).
- Fusion of gametes (fertilization) produces a zygote.
- The zygote grows into the next generation of sporophyte.
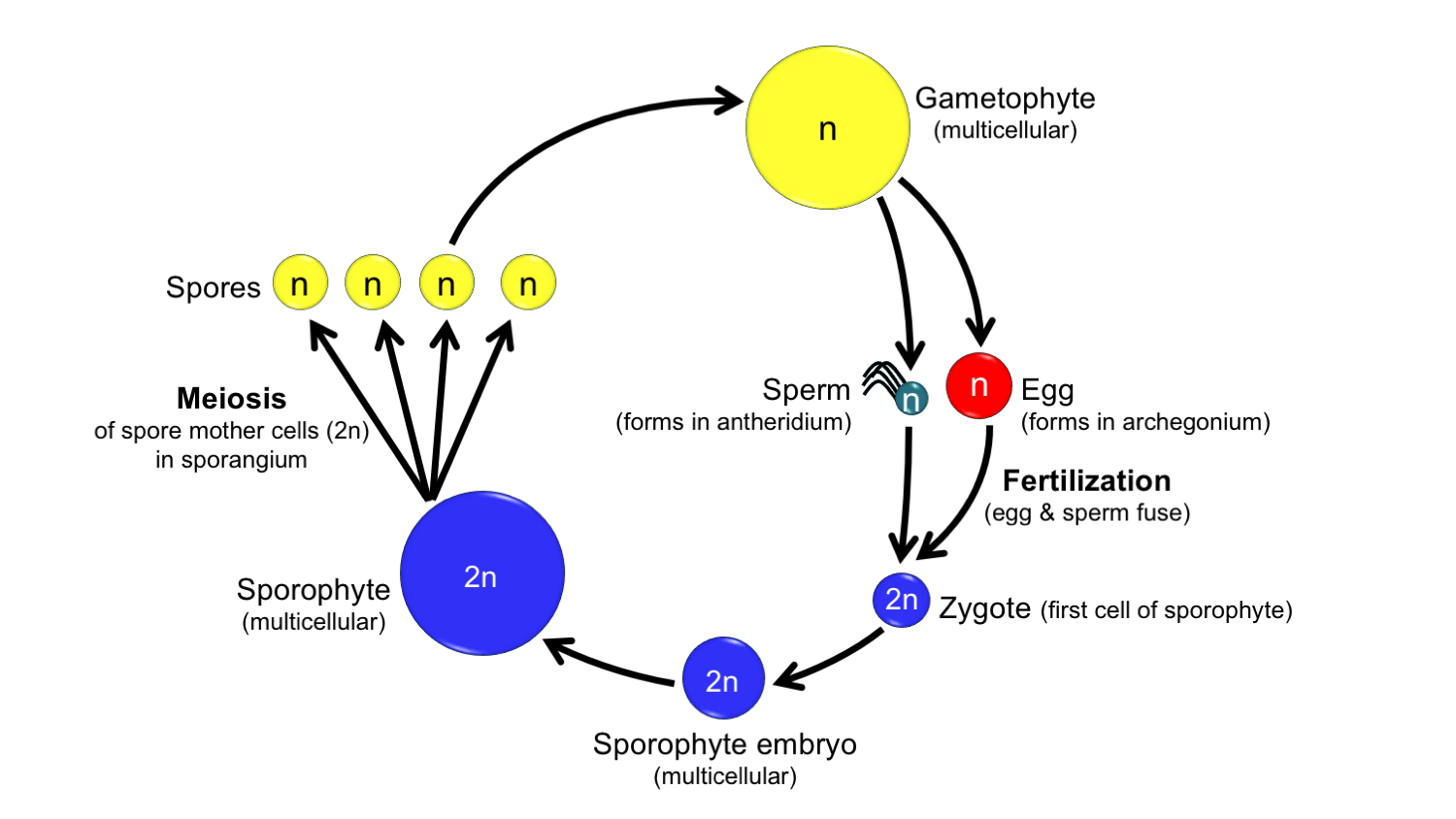
Generalized life cycle of a land plant. Diploid = 2n; haploid = n. Credit: Diagram by E.J. Hermsen (DEAL).
In some plants the gametophyte is the dominant generation, whereas in others the sporophyte is dominant. For example, in a moss, the gametophyte is green and leafy. The simple sporophyte grows on and is dependent on the gametophyte; the sporophyte is typically unbranched and makes only one sporangium during its lifetime.
In a pine, the sporophyte is a tree, whereas the gametophytes are very tiny and strictly unisexual (male or female). The male (sperm-producing) gametophyte is the pollen grain, whereas the female (egg-producing) gametophyte is found in the immature seed (called an ovule). The pollen is released and is carried by wind to the ovule, where it can deliver sperm so that fertilization can occur. The ovule is released from the pine cone sometime after fertilization, when it has matured into a seed containing a sporophyte embryo and stored food.
Regardless of which generation may be dominant, the sporophyte and gametophyte generations in land plants are typically very different in structure and appearance. They can thus be described as heteromorphic (Greek, heteros + morphē = different form).
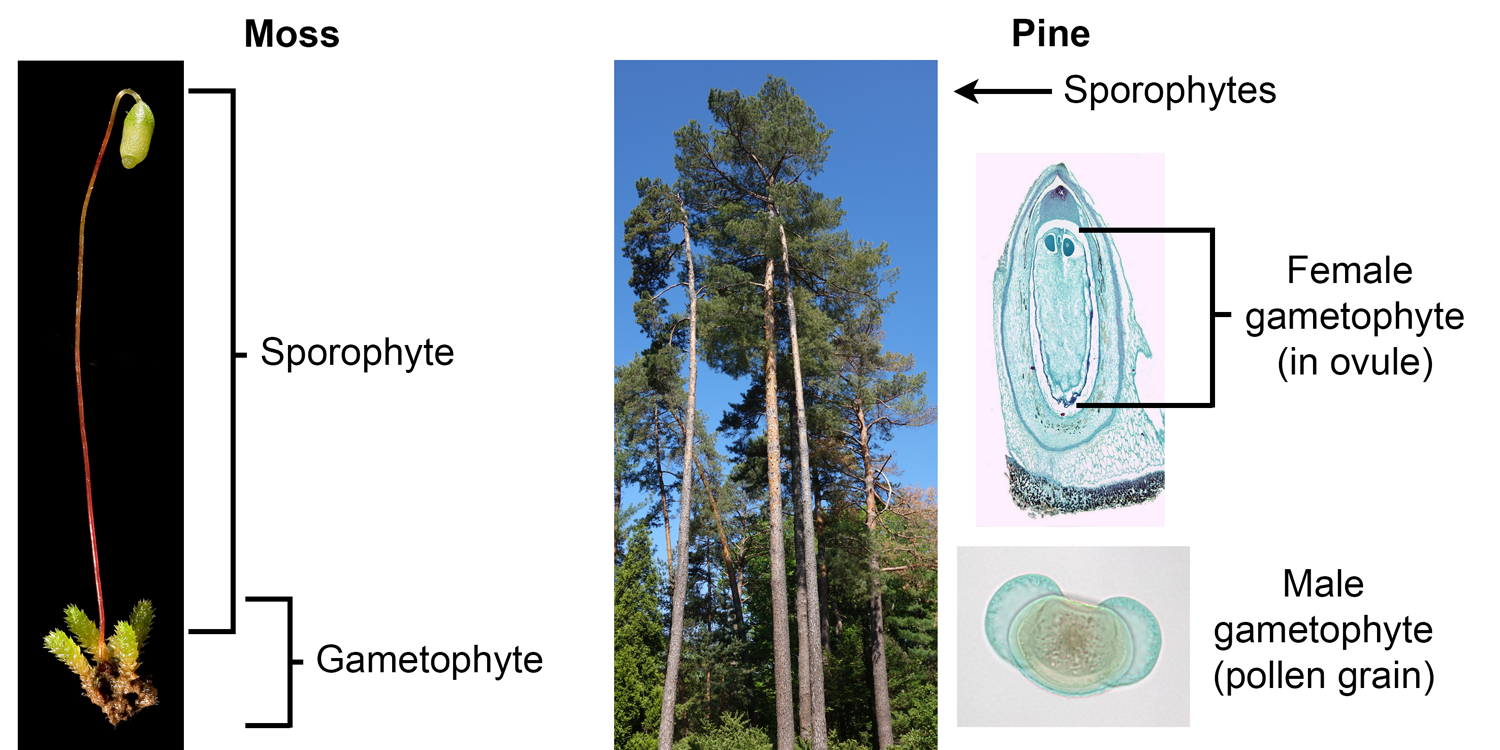
Sporophytes and gametophytes in a moss and a pine. Left: In moss, the gametophyte is the green, leafy plant. Albeit small, it is the dominant generation. The sporophyte is a simple plant with a single, unbranched stalk and a sporangium (capsule) that produces spores. It remains attached to the gametophyte for its entire life. Right: In pine, the sporophyte is the dominant and persistent generation. The gametophytes are, by comparison, very small. The female (egg-producing) gametophyte is in the ovule (immature seed). The male gametophyte is the pollen grain. Image credits: Moss (Kevin Thiele, via flickr, CC BY 2.0); scots pine (Daderot, via Wikimedia Commons, CC0); pine ovule and pollen grain (Jon Houseman & Matthew Ford, via Wikimedia Commons, CC-BY SA 4.0). Images modified from originals.
Homospory & heterospory
In addition to differences in the appearance, persistence, and independence of the sporophyte and gametophyte generations in the life cycles of land plants, there are several other variations that occur in the land plant life cycle. One variation is whether plants are homosporous or heterosporous.
Homospory
Homosporous (from the Greek homos, the same) plants produce one size class of spore, sometimes called isospores (from the Greek isos, equal). Homospory is considered the ancestral condition in land plants.
The gametophytes of homosporous plants are often bisexual, meaning that they produce eggs in archegonia, as well as sperm in antheridia. Such plants have a life cycle like the generalized fern life cycle described above. Sometimes, the gametophytes of these plants may develop as unisexual, or female (archegonia/egg-producing) or male (antheridium/sperm-producing), due to the influence of environmental factors or the production of chemical signals by other nearby gametophytes.
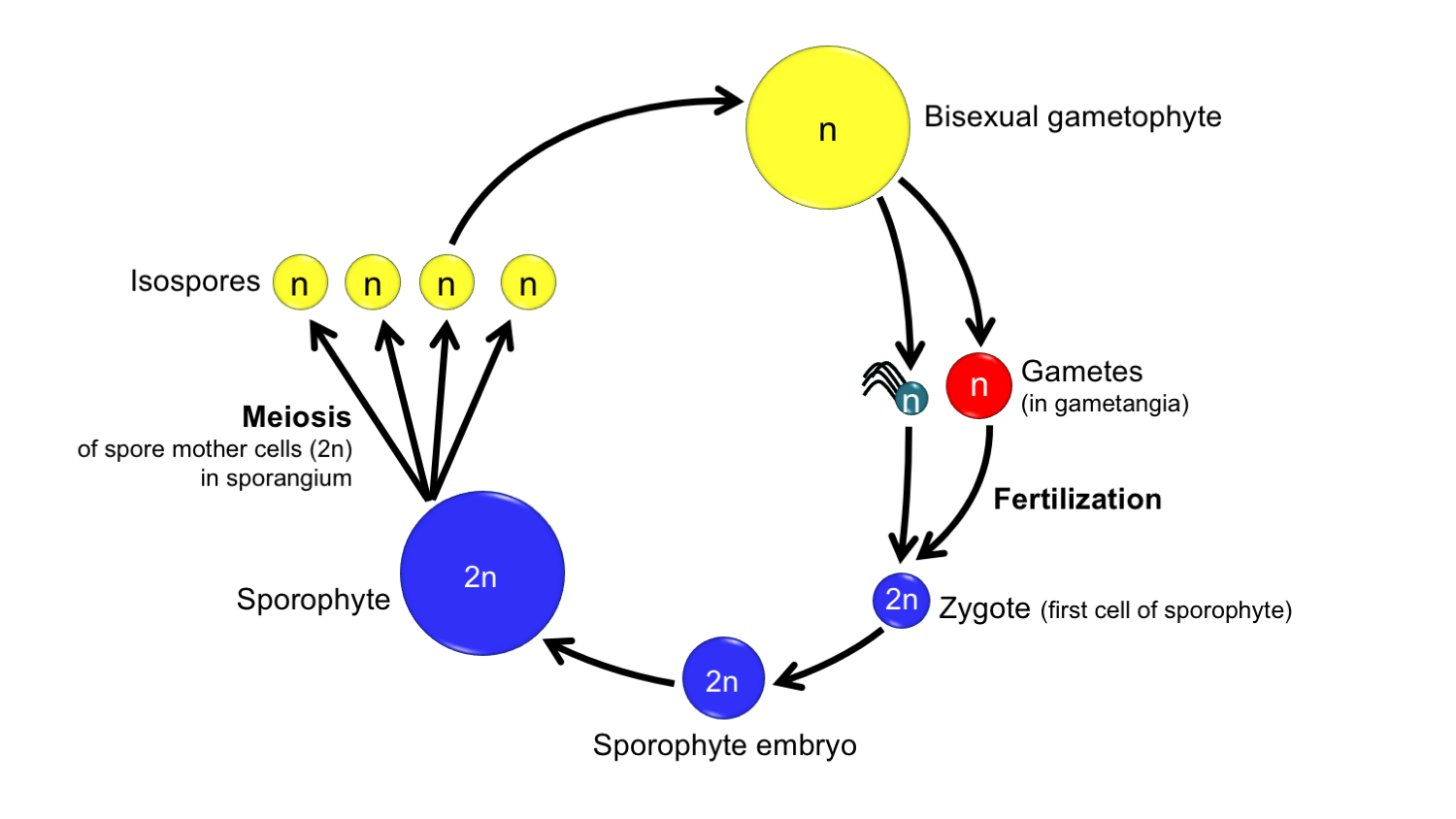
Life cycle of a homosporous plant with bisexual gametophytes. Note that in this life cycle, like that of the fern above, only one type of spore is produced and gametophytes can be bisexual. Credit: Diagram by E.J. Hermsen (DEAL).
Some bryophytes have gametophytes that are unisexual (or dioicous, from the Greek dis + oikos = two houses) due to genetics. These bryophytes have sex chromosomes, often designated U (female) and V (male). Remember that sporophytes are diploid (have two sets of chromosomes) and gametophytes are haploid (have one set of chromosomes). The sporophytes of such plants carry both sex chromosomes. In the simplest cases (like that of umbrella liverwort, shown below), each gametophyte inherits only one sex chromosome from the sporophyte. If a gametophyte inherits a U chromosome, it will be female and produce archegonia with eggs; if it has a V chromosome, it will be male and produce antheridia with sperm.
In the Devonian period, some of the earliest polysporangiophytes (plants with branching sporophytes that produce more than one sporangium) had strictly unisexual gametophytes and may also have had sex chromosomes (read more here).
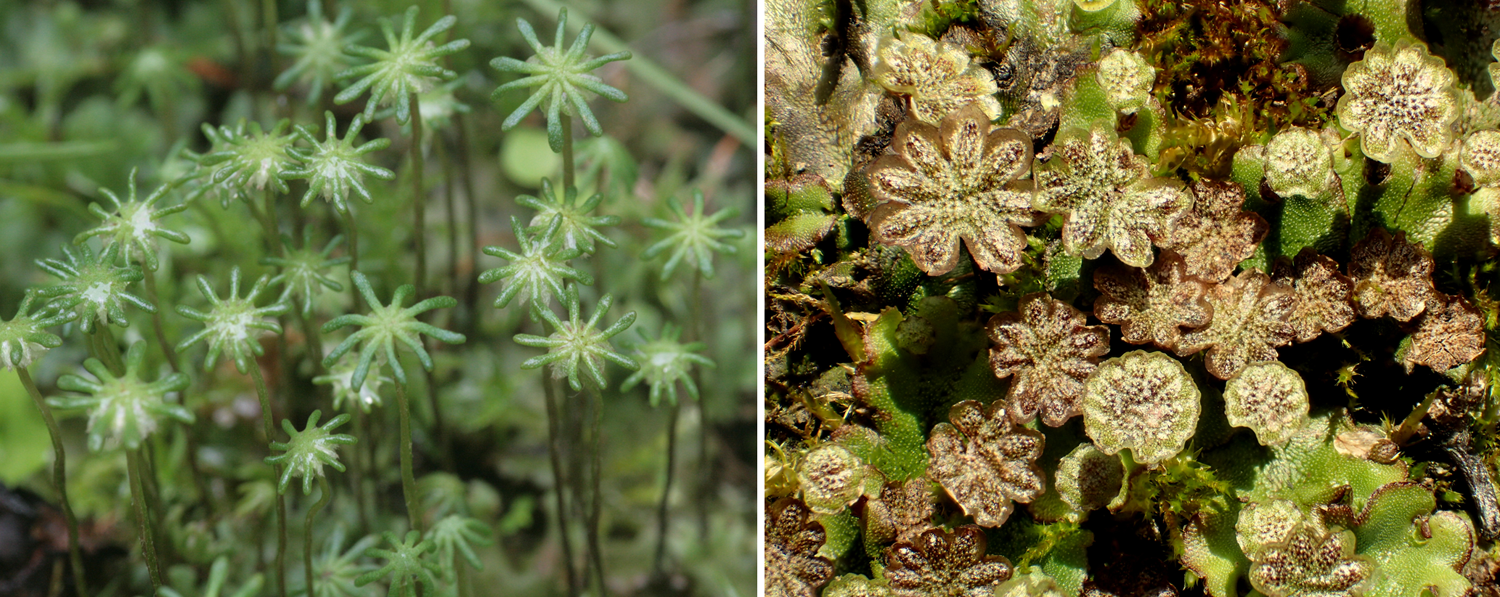
Unisexual gametophytes in a homosporous plant. These are the gametophytes of the umbrella liverwort (Marchantia polymorpha), a model organism that is known to have a U-V (sometimes called X-Y) sex chromosome system. Left: Female gametophyte. The palm-tree-like structures are archegoniophores, or archegonium-bearing structures. The sporophytes develop on the undersides of the heads of the archegoniophores following fertilization. Right: Male gametophytes. The platforms are antheridiophores, or antheridium-bearing structures. When water hits the platforms, sperm are carried away in the droplets formed by the splash. Image credits: Female gametophyte (HermannSchachner, via Wikimedia Commons, CC0); male gametophyte (Brenda Dobbs, via flickr, CC BY-NC 2.0). Images modified from originals.
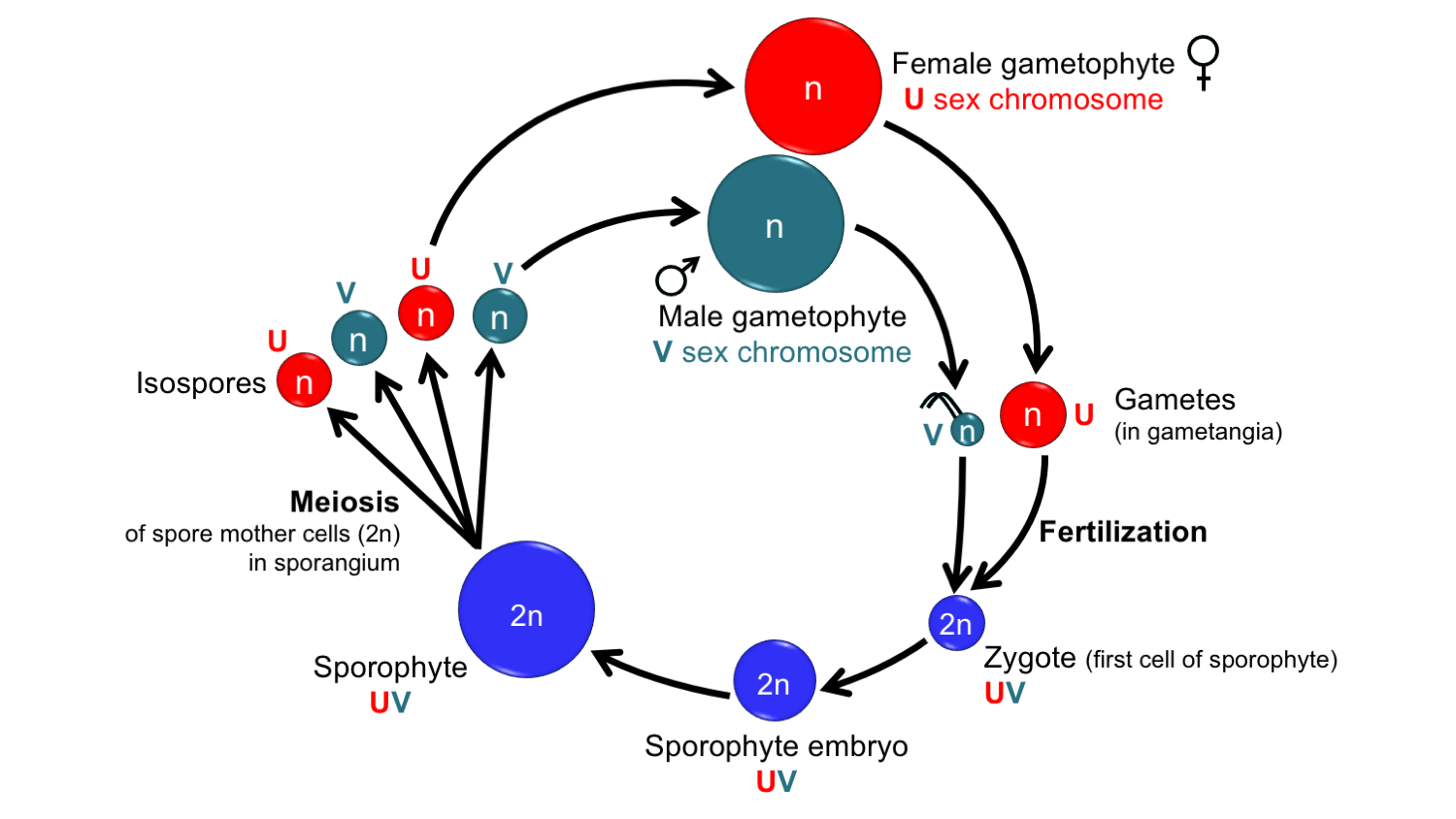
Life cycle of a homosporous plant with sex chromosomes. Note that in this life cycle, the gametophytes are unisexual. The sex of the gametophyte is determined by the sex chromosome that it inherits from the sporophyte, which has both sex chromosomes. The spores of each sex look alike and are mixed together in a common sporangium (contrast to heterosporous plants, below). Note that sometimes "X" is used to denote the female sex chromosome, and "Y" is used to denote the male sex chromosome. Credit: Diagram by E.J. Hermsen (DEAL), after fig. 1 in Renner et al. (2017).
Heterospory
In heterosporous (from the Greek heteros, different) plants, two size classes of spores are produced: larger megaspores and smaller microspores. The prefix "mega-" comes from the Greek word for "large" (megas). In the life cycle of heterosporous plants, "mega-" identifies structures that are associated with production of the female gametophyte. The prefix "micro-" comes from the Greek word for "small" (mikros). In the life cycle of heterosporous plants, "micro-" identifies structures associated with production of the male gametophytes.
The megaspores grow into female (egg-producing) gametophytes, which are called megagametophytes. The microspores grow into male (sperm-producing) gametophytes, which are also known as microgametophytes. The terms "megagametophyte" and "microgametophyte" are used only in heterosporous plants; these terms are not applied to the gametophytes of homosporous plants, even if their gametophytes are unisexual. (The terms "female" and "male" can be applied to unisexual gametophytes of either homosporous or heterosporous plants, and some prefer these terms.)
Production of megaspores and microspores is segregated into separate sporangia. Megaspore mother cells undergo meiosis within megasporangia (megaspore-producing capsules) to produce megaspores. Microspore mother cells undergo meiosis within microsporangia (microspore-producing capsules) to produce microspores. Typically, few megaspores (1 to 4) are produced in each megasporangium, whereas microspores are more numerous in each microsporangium. In some heterosporous plants, megaspores and microspores are produced by separate sporophytes, whereas in other heterosporous plants both types of spores are produced by the same sporophyte.
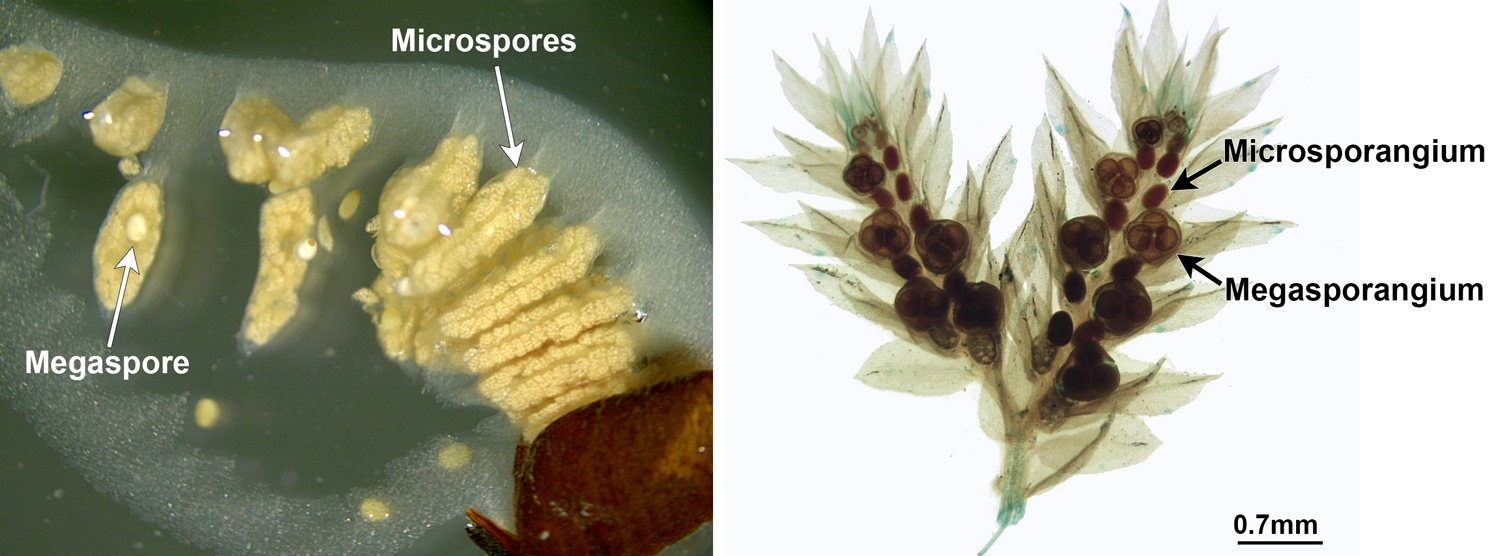
Spores and sporangia in heterosporous plants. Left: Megaspores and microspores being released from the sporocarp of a water clover (Marsilea), a heterosporous fern. Many of the spores are still in their sporangia (capsules), which are translucent. Marsilea has only one megaspore in each megasporangium. Right: Spike moss (Selaginella) strobili (cones) showing megasporangia (each with several megaspores) and microsporangia with microspores. Credits: Marsilea (Curtis Clark, via Wikimedia Commons, CC BY-SA 3.0); Selaginella (Jon Houseman & Matthew Ford, via Wikimedia Commons, CC BY-SA 4.0). Images modified from originals.
The gametophytes of heterosporous plants are very small. They develop entirely or mostly within the confines of the spore wall. In free-sporing (seedless) heterosporous plants, the megaspores and microspores are eventually released from their sporangia. In seed plants, the megaspores are never released from the megasporangium; instead, the megasporangium is a permanent part of the ovule, or immature seed (read more about seed plants below).
While the details differ, the life cycles of heterosporous plants follow these basic steps:
- The sporophyte produces megaspores in megasporangia and microspores in microsporangia. (Remember, spores are produced by meiosis.)
- The megaspores grow into megagametophytes, whereas the microspores grow into microgametophytes.
- The megagametophytes produce eggs, whereas the microgametophytes produce sperm.
- Fusion of gametes (fertilization) produces a zygote.
- The zygote grows into the next generation of sporophyte.
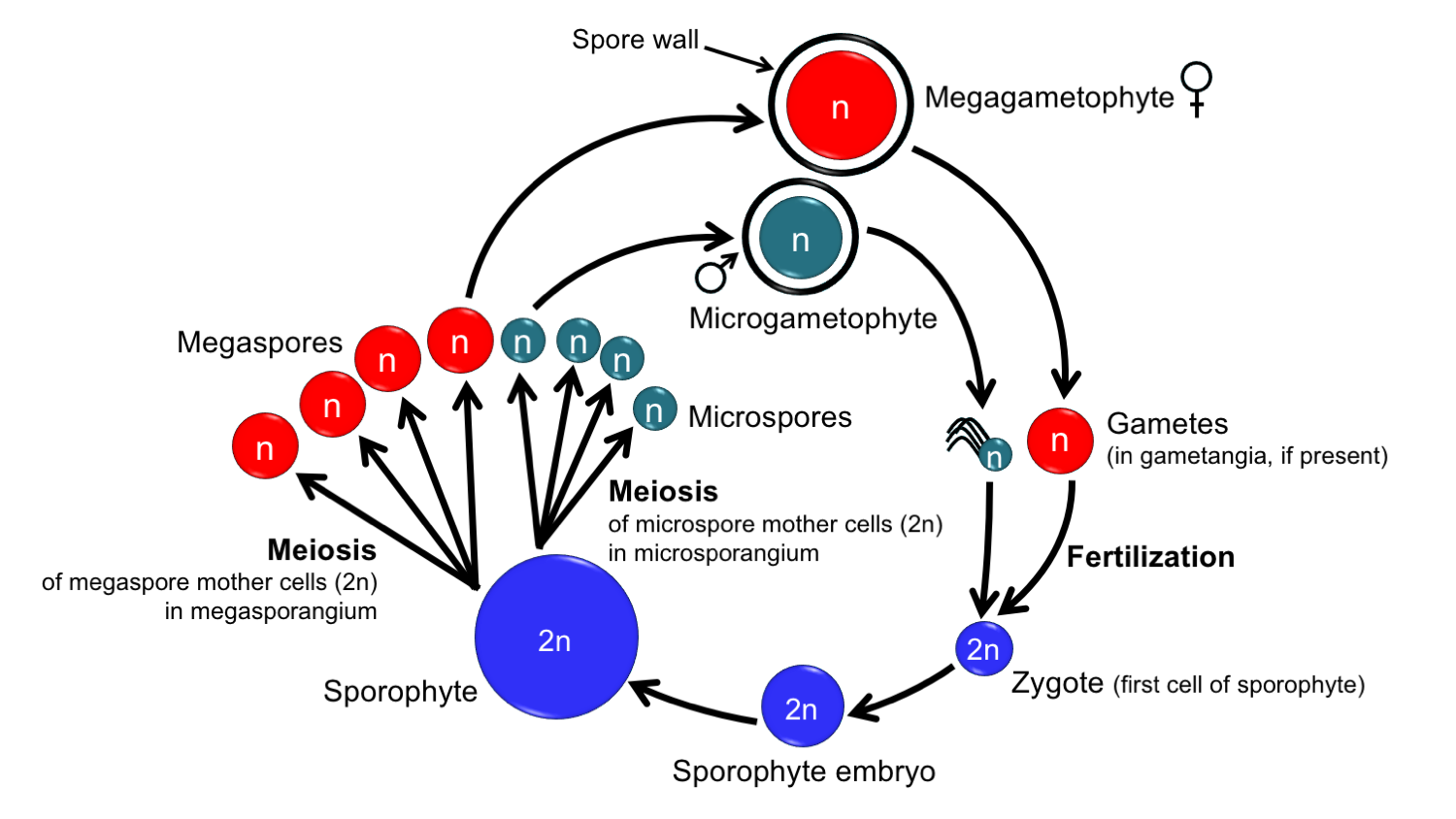
Generalized life cycle of a heterosporous plant. In seed plants, the pollen grains (microgametophytes) do not produce antheridia. The megagametophytes sometimes have archegonia and sometimes do not. Credit: Diagram by E.J. Hermsen (DEAL).
Homosporous Plants vs. Heterosporous Plants
The table below compares the distinguishing characteristics of homosporous and heterosporous plants.
Homosporous plants | Heterosporous plants |
---|---|
Isospores (spores cannot be divided into two distinct size classes) | Megaspores (larger spores) and microspores (smaller spores) |
All sporangia similar; spores not sorted into different types of sporangia | Megasporagia produce megaspores, microsporangia produce microspores |
Gametophytes unisexual (female or male, not both) or bisexual (both eggs and sperm produced on same individual) | Gametophytes unisexual; megagametophytes (female gametophytes) develop from megaspores, microgametophytes (male gametophytes) develop from microspores |
Gametophytes exosporic (= live outside the confines of the spore wall) | Gametophytes endosporic (= confined mostly or entirely within the spore wall) |
Living land plant groups: bryophytes (non-vascular plants), clubmosses (Lycopodiaceae), most ferns (Marattiales, Ophioglossales, Psilotales, most leptosporangiate orders), horsetails (Equisetum) | Living land plant groups: quillworts (Isoetes), spikemosses (Selaginella), water ferns (Salviniales), seed plants |
Dispersed megaspores and differently-sized spores preserved in the sporangia of fossil plants provide evidence for the origin of heterospory in the Early to Middle Devonian (see, e.g., here and here). Heterospory has evolved from homospory multiple times within land plants, making this life cycle switch an important part of understanding plant macroevolution. In fact, heterospory has been called "the most iterative [repeated] key innovation" in land plant macroevolution.
Heterospory is known in a few living plant groups: some lycophytes (Isoetes and Selaginella), water ferns (Salviniales), and the seed plants. It also occurred in some extinct plants, like the ancient scale trees (an extinct group of large lycophytes) of the Carboniferous Period and some extinct relatives of the modern horsetails (Equisetum). Megaspores form an important part of the plant fossil record and provide clues to the evolution of certain plant groups, especially in the Paleozoic and Mesozoic eras.
The seed habit
Seed plants first appeared in the Late Devonian (see, e.g., here and here). All plants that produce seeds are heterosporous plants, so the seed habit involves further modifications beyond simple heterospory. These include the evolution of the ovule and seed, as well as the pollen grain.
A seed is a package that is made up of a protective seed coat that surrounds a sporophyte embryo and stored food that the young sporophyte can use early in its growth. A seed develops from an ovule. An ovule is a structure that consists of megasporangium (called a nucellus in seed plants) that is surrounded by one or two envelopes of tissue called integuments. A single diploid megaspore mother cell develops with the megasporangium (nucellus). Meiosis of the megaspore mother cell typically yields one functional haploid megaspore; the other three megaspores typically degenerate, or break down.
The nucellus never opens to release the megaspore inside. Instead, the megaspore grows into a tiny megagametophyte within the nucellus. The megagametophyte may produce one or more eggs, depending on the type of plant. In some seed plants, the eggs are produced in archegonia, whereas in others (most notably, the flowering plants) the megagametophytes are very simple and the archegonia are no longer formed.
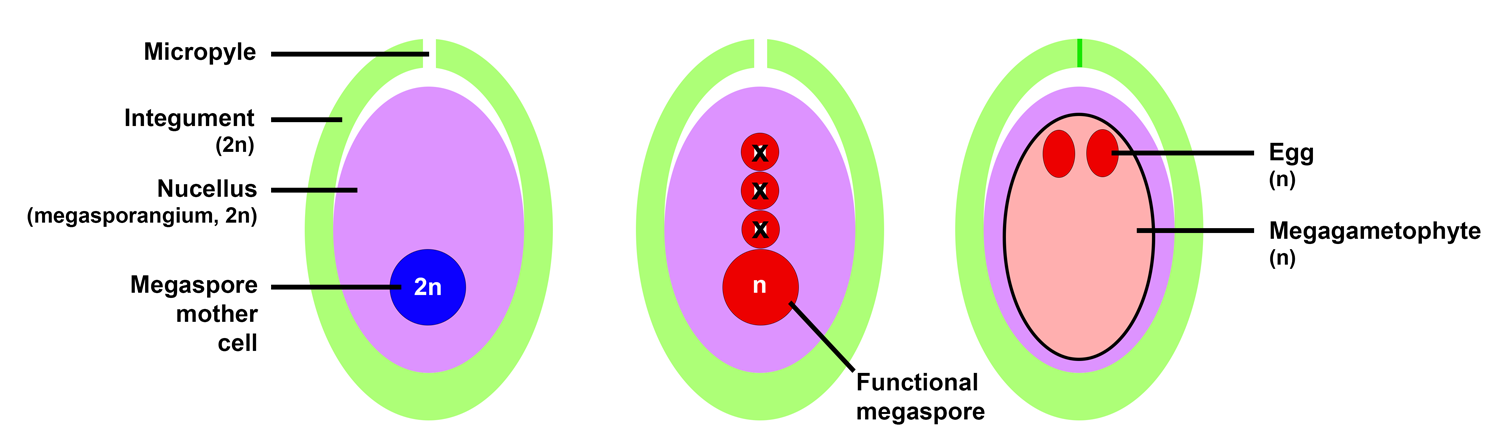
Typical ovule development in a gymnosperm (non-flowering seed plant). Left: Ovule with megaspore mother cell. Center: Ovule following meiosis. Four megaspores are produced. Three megaspores usually degenerate, whereas one is functional; the functional megaspore begins dividing and developing into a megagametophyte. Right: The multicellular megagametophyte with one or more eggs develops within the megasporangium (nucellus). Credit: Diagram by E.J. Hermsen (DEAL).
The microgametophyte in seed plants is called a pollen grain. Pollen grains begin their development in the microsporangia, which are sometimes called pollen sacs in seed plants. Within the microsporangia, diploid microspore mother cells (also called pollen mother cells) form. Each microspore mother cell undergoes meiosis to produce a tetrad, or group of four, haploid microspores. Each microspore then begins dividing to form a tiny microgametophyte, the pollen grain. The pollen grain is surrounded by a pollen wall. The pollen sacs open to release the pollen grains, which may be carried away by the wind, animals, or other vectors so that pollination can occur.
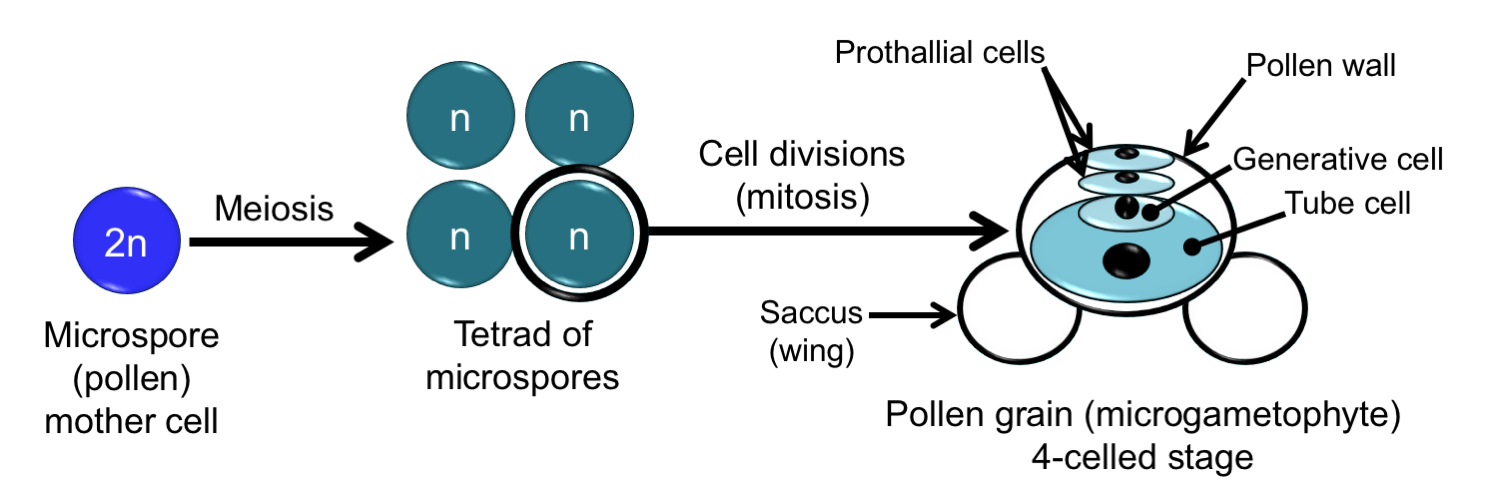
Pollen development in pine (Pinus). Pollen development in seed plants begins with microspore mother cells (pollen mother cells) that differentiate in the microsporangia (pollen sacs). Each of the microspore mother cells undergoes meiosis to produce a tetrad (group of four) microspores. Each microspore then divides to form a pollen grain. Details of pollen grain development in seed plants differ after the microspore stage. In pine, the pollen grain has four cells when it is released from its microsporangium (pollen sac). It is surrounded by a pollen wall with sacci (bladders or "wings") that aid in wind dispersal. The tube cell will grow through the pollen wall to form a pollen tube after pollination. The generative cell is the grandparent of the sperm cells. The prothallial cells eventually degenerate. Credit: Diagram by E.J. Hermsen (DEAL).
In gymnosperms (non-flowering seed plants), the pollen grain lands on the tip of the ovule and enters it through a hole in the integument called the micropyle. This process may be facilitated by a pollination drop, a bead of liquid exuded from the micropyle. Once inside the ovule, the pollen grain germinates and matures. Because the ovules of angiosperms (flowering plants) are enclosed in another structure, the ovary, pollen grains cannot land directly on the ovules. Instead, they land on a specialized surface outside the ovary, the stigma, where they germinate.
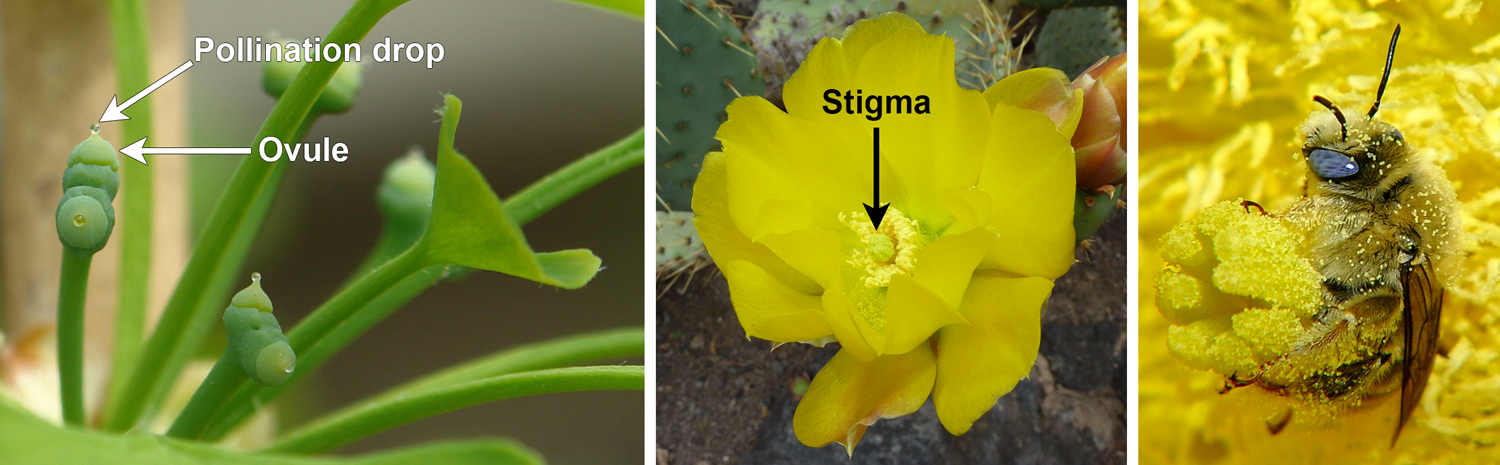
Examples of pollen-receiving structures in seed plants. Left: Ovules of ginkgo (Ginkgo biloba), a gymnosperm. Each ovule has a pollination drop that is exuded from the micropyle (opening) in the integument at the tip of the ovule. Center: Flower of cactus apple (Opuntia engelmannii), an angiosperm. The stigma sticks out above the ring of stamens in the center of the flower. Right: Detail of stigma of cactus apple (O. engelmannii) dusted with pollen. Image credits: ginkgo (Marcin Kolasiński, via Wikimedia Commons, CC BY-SA 4.0); cactus flower (James Steakley, via Wikimedia Commons, CC BY-SA 3.0); stigmas (Jessie Eastland, via Wikimedia Commons, CC BY-SA 4.0).
Although the terms are sometimes used interchangeably, pollination (transfer of pollen grains from microsporangia to ovules or stigmas) and fertilization (fusion of sperm and egg) are separate processes in seed plants. Pollen grains often mature and produce their sperm following pollination. The mature pollen grain is very simple; it does not have an antheridium and typically produces only two sperm. The details of fertilization differ in different kinds of seed plants, although they can be divided into two major groups: those that have motile (swimming) sperm, and those that do not.
- Zoidogamous (Greek, zoion + gamia = motile union; also spelled zooidogamous) seed plants have motile (swimming) sperm, like seedless plants. In zoidgamous seed plants, the pollen grain will eventually release its sperm inside the ovule so that they can swim the short distance to the egg(s).
- Siphonogamous (Greek, siphon + gamia = tube union) seed plants have nonmotile sperm, or sperm that do not swim. In siphonogamous seed plants, the sperm are delivered directly to an egg via an extension of the pollen grain called the pollen tube.
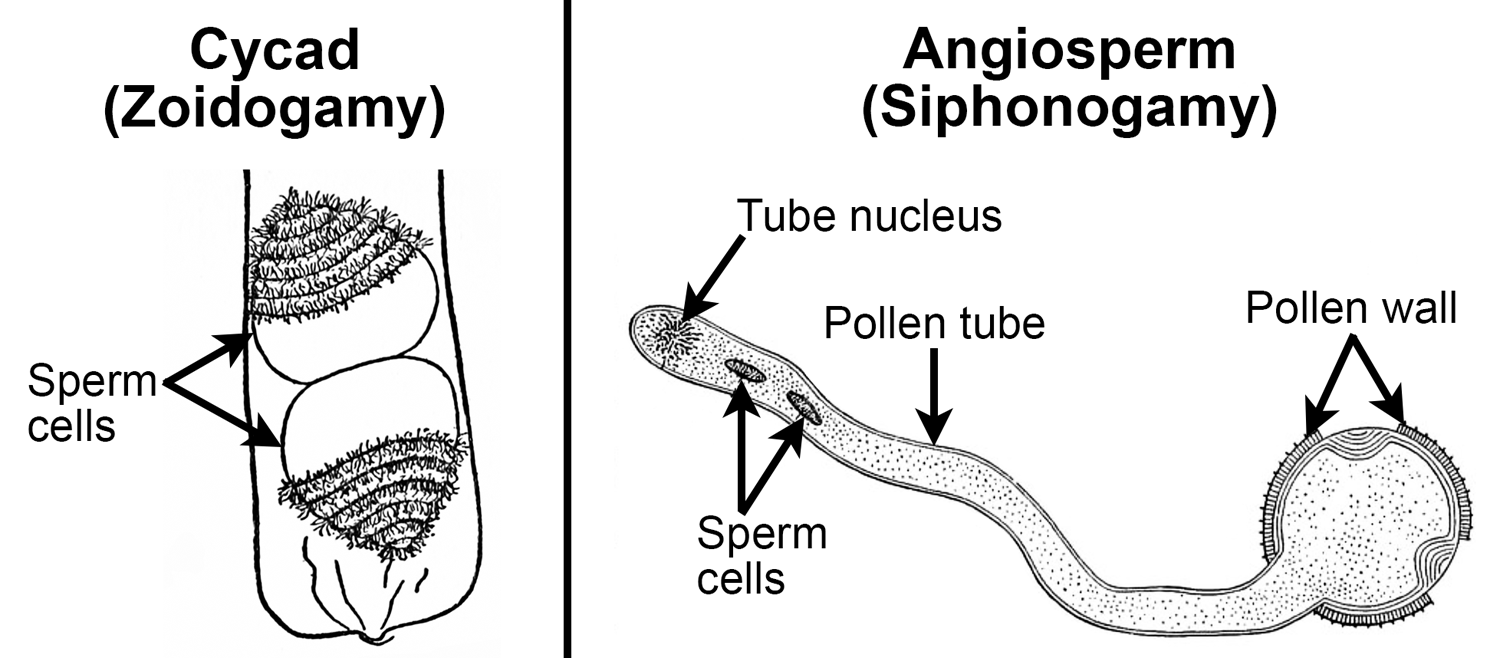
Zoidogamy vs. siphonogamy in seed plants. Left: The multiflagellated sperm cells of a cycad. The sperm cells are depicted within the pollen grain. The pollen grain ruptures to release them within the ovule. Right: A germinated angiosperm pollen grain with pollen tube. Note the degenerating tube nucleus and two sperm cells. Credits: Drawing of cycad sperm, fig. 7B from Scott (1911) Evolution of Plants (no known copyright restrictions); Drawing of germinated dicot pollen grain, fig. 118 from Bergen & Caldwell (1914) Introduction to Botany (no known copyright restrictions). Images modified from originals.
The time that elapses between pollination and fertilization varies enormously, from less than 24 hours to over a year in different groups of seed plants. In seed plants where months elapse between pollination and fertilization, the pollen grain germinates inside the ovule and absorbs food from a fleshy nucellus (megasporangium).
Once an egg is fertilized to form a zygote, the zygote will begin dividing to develop into an sporophyte embryo. The integument(s) of the ovule will harden to become the seed coat. Often, food will be present as a separate tissue. In gymnosperm seeds, the stored food in the seed is the megagametophyte itself. In angiosperm seeds, a specialized food tissue called endosperm (Greek, endon + sperma = within seed) is formed. The seed coat and its enclosed embryo, along with any stored food, is now a seed. Eventually, the seed will germinate and the young sporophyte will resume its growth and develop into a mature plant.
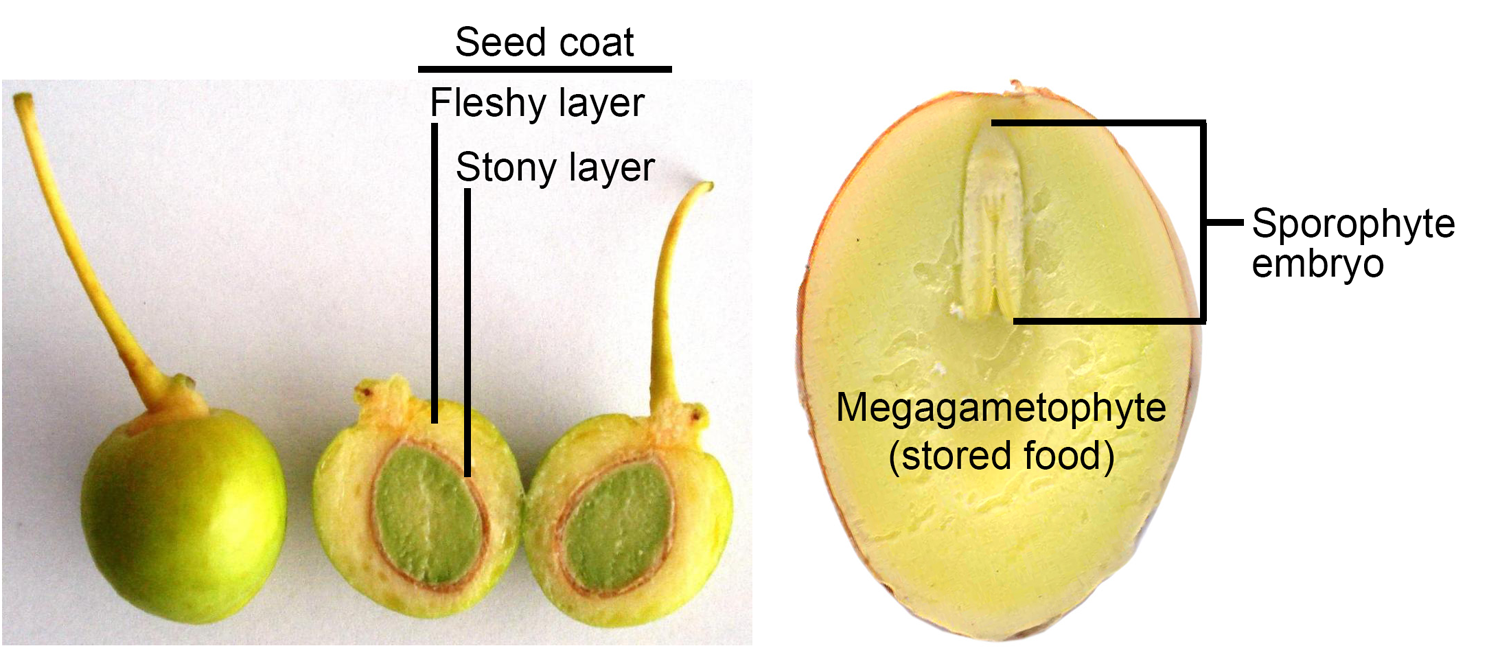
Ginkgo (Ginkgo biloba) seeds. Left: Immature whole ginkgo seed next to a ginkgo seed cut lengthwise. The seed coat of ginkgo has an outer fleshy layer (the stinky layer) and an inner hard layer. The green megagametophyte is in the center of the seed. Right: Ginkgo seed with seed coat removed, cut lengthwise. The inside of the seed consists of fleshy megagametophyte tissue (now serving as stored food for the embryo) and a tiny sporophyte embryo. Note that the base of the embryo is at the apex (top) of the seed. The structures that look like rabbit ears are the seed leaves (cotyledons) of the embryo. Credits: Ginkgo biloba seeds (Genet, via Wikimedia Commons, CC BY-SA 3.0); Long section of ginkgo seed (Gmihail, via Wikimedia Commons, CC BY-SA 3.0 RS).
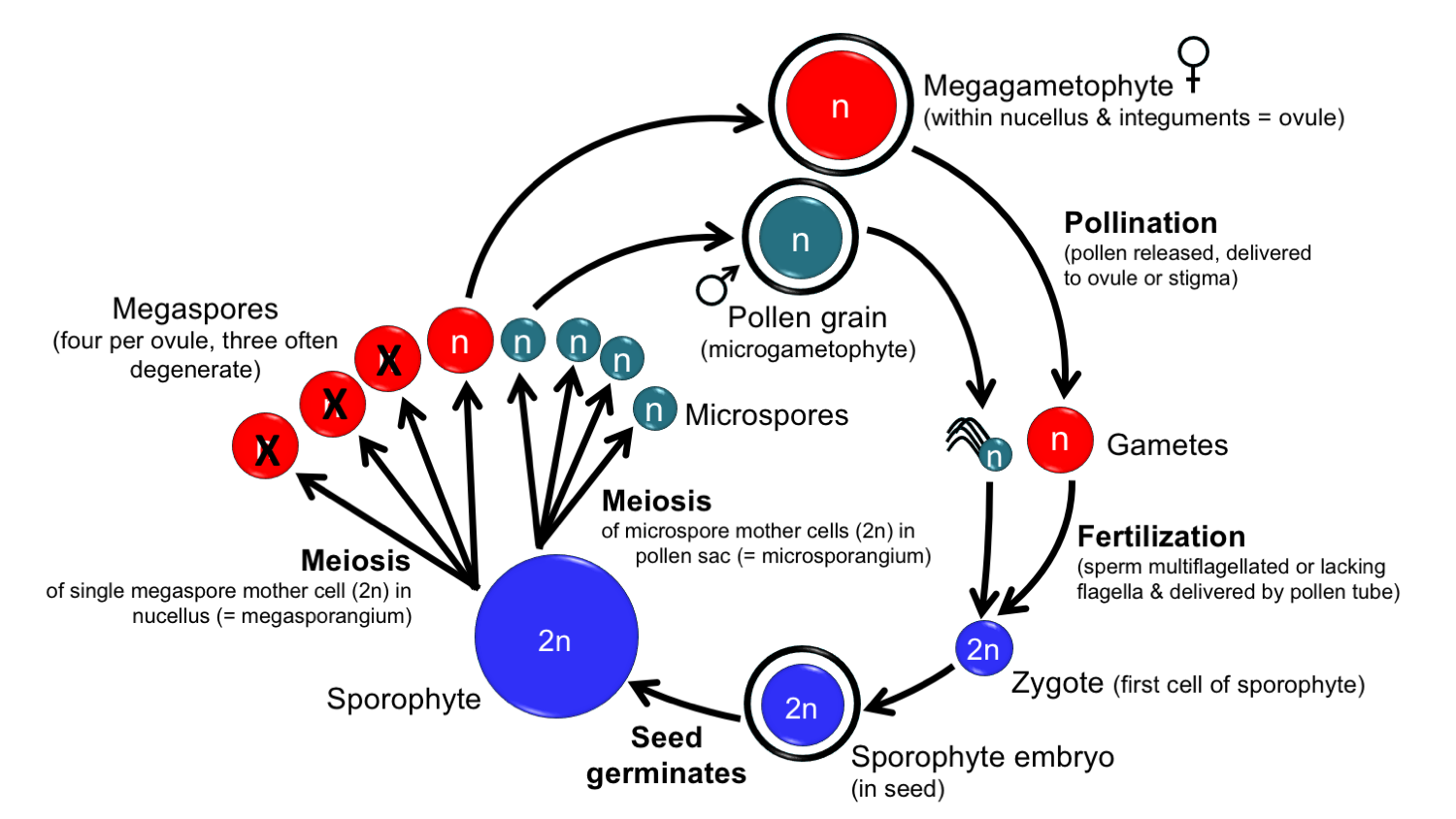
Generalized life cycle of a seed plant. In seed plants, the pollen grains (microgametophytes) do not produce antheridia. The megagametophytes sometimes have archegonia and sometimes do not. Specifically, archegonia are not produced in Gnetum, Welwitschia, and the flowering plants. Generally speaking, the gametangia were lost during evolutionary simplification of the gametophytes. Credit: Diagram by E.J. Hermsen (DEAL).
The pollen grain, the ovule, and the seed provide important advantages to plants in completing their life cycles on land. In seedless plants, which all have motile sperm, the sperm require the presence of water in order to swim to the egg. In seed plants, the pollen grain delivers the sperm to the egg within the ovule, and no additional water is needed. Furthermore, the pollen grain can travel to an ovule (or stigma) within the protective pollen wall, so individual plants need not be in extremely close proximity in order to complete their life cycles.
In seedless plants, the young sporophyte must grow and develop from zygote to embryo to mature plant without an extended period of rest. In seed plants, the sporophyte embryo begins its development in the protective environment supplied by the ovule. Once the seed is mature, the young sporophyte can survive while awaiting the proper conditions for germination. Seeds may remain in the soil for days, months, or years before germinating; in a few cases, researchers have been able to successfully germinate seeds that are over 1,000 years old (see here and here)! When growth begins again, the seed contains food to sustain the young sporophyte until it can begin photosynthesizing to sustain itself.
Selected references & further reading
Note: Free full text is made available by the publisher for items marked with a green star.
Academic articles
* Andrews, H.N., P.G. Gensel, and W.H. Forbes. 1974. An apparently heterosporous plant from the Middle Devonian of New Brunswick. Palaeontology 17: 387-408. https://www.palass.org/publications/palaeontology-journal/archive/17/2/article_pp387-408
Bateman, R.M., and W.A. DiMichele. 1994. Heterospory: The most iterative key innovation in the evolutionary history of the plant kingdom. Biological Reviews 69: 345-417. https://doi.org/10.1111/j.1469-185X.1994.tb01276.x
Coelho, S.M., J. Gueno, A.P. Lipinska, J.M. Cock, and J.G. Umen. 2018. UV chromosomes and haploid sexual systems. Trends in Plant Science 23: 794-807. https://doi.org/10.1016/j.tplants.2018.06.005
* Qiu, Y.-L., A.B. Taylor, and H.A. McManus. 2012. Evolution of the life cycle in land plants. Journal of Systematics and Evolution 50: 171–194. https://doi.org/10.1111/j.1759-6831.2012.00188.x
* Renner, S.S., J. Heinrichs, and A. Sousa. 2017. The sex chromosomes of bryophytes: Recent insights, open questions, and reinvestigations of Frullania dilatata and Plagiochila asplenioides. Journal of Systematics and Evolution 55: 333-339. https://doi.org/10.1111/jse.12266
Rothwell, G.W., S.E. Scheckler, and W.H. Gillespie. 1989. Elkinsia gen. nov., a Late Devonian gymnosperm with cupulate ovules. Botanical Gazette 150: 170-189. https://doi.org/10.1086/337763
Sallon, S., E. Solowey, Y. Cohen, R. Korchinsky, M. Egli, I. Woodhatch, O. Simchoni, and M. Kislev. 2008. Germination, genetics, and growth of an ancient date seed. Science 320: 1464. https://doi.org/10.1126/science.1153600
Shen-Miller, J., M.B. Mudgett, J.W. Schopf, S. Clarke, and R. Berger. 1995. Exceptional seed longevity and robust growth: ancient sacred lotus from China. American Journal of Botany 82: 1367-1380. https://doi.org/10.1002/j.1537-2197.1995.tb12673.x
* Shimamura, M. 2016. Marchantia polymorpha: Taxonomy, phylogeny and morphology of a model system. Plant & Cell Physiology 57: 230-256. https://doi.org/10.1093/pcp/pcv192
Steemans, P., P. Breuer, E. Petus, C. Prestianni, F. de Ville de Goyet, P. Gerrienne. 2011. Diverse assemblages of Mid Devonian megaspores from Libya. Review of Palaeobotany and Palynology 165: 154-174. https://doi.org/10.1016/j.revpalbo.2011.02.007
* Taylor, T.N., H. Kerp, and H. Hass. 2005. Life history biology of early land plants: Deciphering the gametophyte phase. Proceedings of the National Academy of Sciences of the United States of America 102: 5892-5897. https://doi.org/10.1073/pnas.0501985102
* Tanurdzic, M., and J.A. Banks. 2004. Sex-determining mechanisms in land plants. The Plant Cell 16: S61-S71. https://doi.org/10.1105/tpc.016667
* Wang, D.-M., J.F. Basinger, P. Huang, L. Liu, J.-Z. Xue, M.-C. Meng, Y.-Y. Zhang, and Z.-Z. Deng. 2015. Latisemenia longshania, gen. et sp. nov., a new Late Devonian seed plant from China. Proceedings of the Royal Society B, Biological Sciences 282: 20151613. https://doi.org/10.1098/rspb.2015.1613
Books & textbooks
Bergen, J.Y., and O.W. Caldwell. 1914. Introduction to Botany. Ginn and Company, Boston. Read online at the Internet Archive.
Evert, R.F., and S.E. Eichhorn. 2013. Raven Biology of Plants, 8th ed. W.H. Freeman and Co., New York, New York.
Foster, A.S., and E.M. Gifford, Jr. 1974. Comparative Morphology of Vascular Plants, 2nd ed. W.H. Freeman and Co., San Francisco.
Simpson, M.G. 2010. Plant Systematics, 2nd ed. Academic Press, Burlington, Massachusetts.
Scott, H.D. 1911. The Evolution of Plants. Henry Holt and Co., New York, and Williams and Norgate, London. Read online at the Internet Archive.
Content usage
Usage of text and images created for DEAL: Text on this page was written by Elizabeth J. Hermsen. Original written content created by E.J. Hermsen for the Digital Encyclopedia of Ancient Life that appears on this page is licensed under a Creative Commons Attribution-NonCommercial-ShareAlike 4.0 International License. Original images and diagrams created by E.J. Hermsen are also licensed under Creative Commons Attribution-NonCommercial-ShareAlike 4.0 International License.
Content sourced from other websites: Attribution, source webpage, and licensing information or terms of use are indicated for images sourced from other websites in the figure caption below the relevant image. See original sources for further details. Attribution and source webpage are indicated for embedded videos. See original sources for terms of use. Reproduction of an image or video on this page does not imply endorsement by the author, creator, source website, publisher, and/or copyright holder.
Adapted images. Images that have been adapted or remixed for DEAL (e.g., labelled images, multipanel figures) are governed by the terms of the original image license(s) covering attribution, general reuse, and commercial reuse. DEAL places no further restrictions above or beyond those of the original creator(s) and/or copyright holder(s) on adapted images, although we ask that you credit DEAL if reusing an adapted image from the DEAL website. Please note that some DEAL figures may only be reused with permission of the creator(s) or copyright holder(s) of the original images. Consult the individual image credits for further details.
Last updated: 13 June 2020.