Feature image: Ginkgo (Ginkgo biloba) leaves that were shed from trees in the fall. Ginkgo leaves have dichotomous venation, or a pattern of venation in which the veins fork one or more times. Credit: E.J. Hermsen (DEAL).
Topics covered on this page:
Introduction
Leaves are part of the shoot system of the vascular plant sporophyte and one of the three major vegetative (non-reproductive) organs types found in vascular plants (the others are stems and roots). The primary function of leaves is to carry out photosynthesis. Photosynthesis is the process by which a plant makes its food. The plant produces carbohydrates (sugars) using carbon dioxide (CO2) and water (H2O) molecules; as is well known, oxygen (O2) is also produced during photosynthesis. Sunlight provides the energy to make this happen. Leaves are often thin and flat to maximize their ability to intercept sunlight.
While leaves often function in photosynthesis, they can be modified to play many other roles. As spines, they are sharp, pointy defensive structures. As tendrils, they provide support for climbing. Leaves may also be non-photosynthetic and modified for food storage. In low-nutrient environments, some carnivorous plants have leaves that have been modified to capture prey.
Leaves have evolved multiple times during the course of plant evolution. Thus, the leaf of a firmoss (Huperzia, a type of lycophyte and not a true moss) has a different evolutionary origin than the leaf of a tree fern, which has a different evolutionary origin than the leaf of a cycad (a gymnosperm).
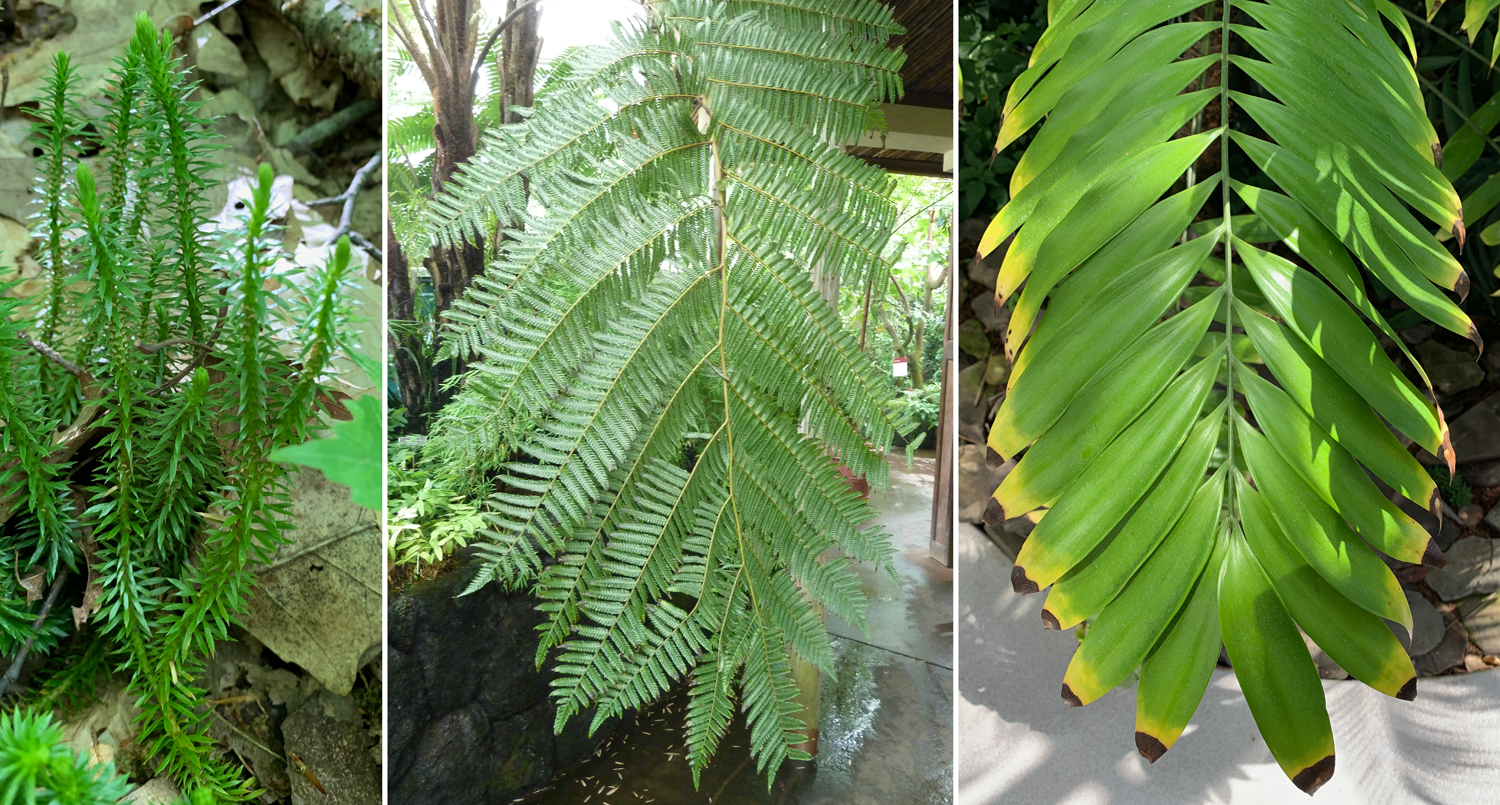
Leaves that have separate evolutionary origins. Left: Shining firmoss (Huperzia lucidula, a lycophyte) with small, simple leaves. Center: Portion of a complex tree fern frond (Alsophila firma). Right: Portion of a pinnately compound cycad (Zamia sp.) leaf. Credits: All images by E.J. Hermsen (DEAL).
Structural definition of the leaf
If leaves cannot be clearly defined according to function or according to a single evolutionary origin, what makes a leaf a leaf? One way to define a leaf is on the basis of its structural characteristics. Although no one structural feature clearly defines a leaf, leaves tend to exhibit all or most of the following features:
- Lateral appendages: Leaves are borne laterally on stems (in other words, leaves occur on the sides of stems).
- Exogenous/superficial development: Leaves begin development superficially, or on the surface of the stem; they first emerge at the shoot apex.
- Phyllotaxy: Leaves occur in a regular arrangement on the stem (Greek phyllon + taxis = leaf arrangement).
- Vascularization: A leaf has one or more strands of vascular tissue, or tissue that conducts food (phloem) and water (xylem).
- Determinate growth: Leaves usually grow for a limited period of time, reach a final form, and then stop growing.
- Dorsiventrality: The upper and lower regions of a leaf often differ in their anatomy (internal structure).
- Bilateral symmetry/lack of radial symmetry: Leaves are often planar (flattened) structures; leaves have length and width, but are relatively thin. In contrast, stems and roots are often roughly cylindrical and appear circular in cross section.
Of course, we usually do not have to use the above characteristics to determine whether a structure is a leaf, as in most cases identifying leaves is pretty straightforward. Rather, the above characteristics are important to keep in mind when studying the origins of leaves, as well as in evaluating some difficult cases involving ambiguous organs in plants.
Leaf morphology
Leaves come in a wide variety of sizes and forms across vascular plants, from the scale leaves of some conifers to enormous, broad leaves of some flowering plants (like Coccoloba gigantifolia, shown here) to the highly divided leaves of the tree fern Cyathea (see here). The stereotypical leaf consists of a wide, flat, green blade or lamina attached to the stem by a stalk called a petiole. This type of leaf is a called a simple leaf, because the lamina or blade is undivided. The base of the lamina is the end closest to the stem, whereas the apex is the end furthest from the stem. The edge of the lamina is known as the leaf margin. The vascular bundles (in other words, the strands of tissue that conduct food and water) in the leaf blade are also known as the veins.
The leaf blade has an upper surface, called the adaxial side, and a lower surface, called the abaxial side. Technically, "adaxial" and "abaxial" refer to the side of the leaf relative to the axis, or stem; adaxial (Greek ad = to, toward) indicates the side facing the stem, abaxial (Greek ab = away, from) indicates the side facing away from the stem. The direction of the leaf nearer the stem is the basal or proximal direction, whereas the direction further from the stem is the apical or distal direction.
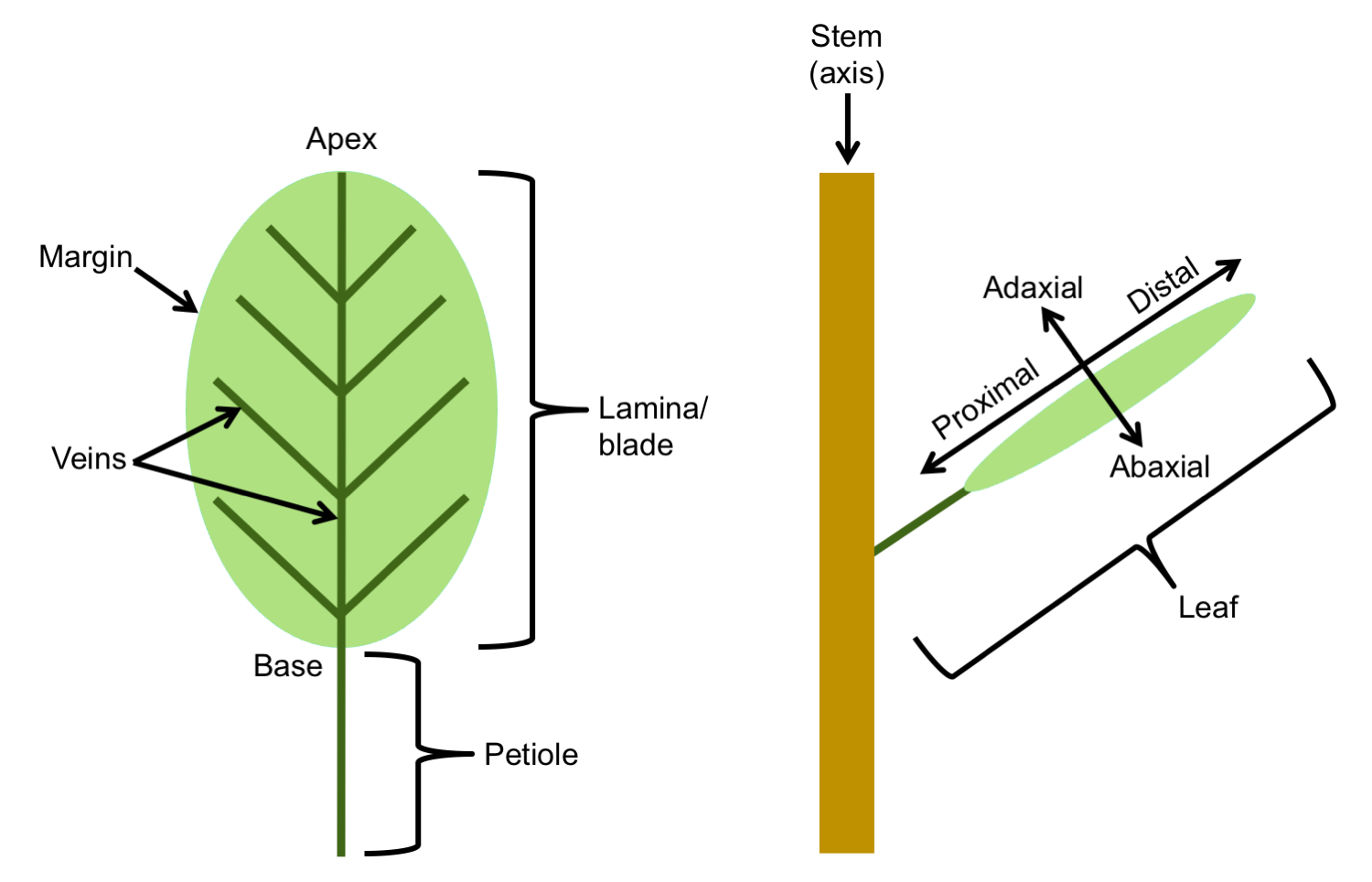
Leaf parts and directional terms. Left: Diagram of a simple leaf showing the basic parts, including the petiole (stalk), lamina (blade), veins (strands of vascular tissue), margin (edge of the lamina), apex of the lamina, and base of the lamina. Right: Diagram of a leaf attached to a stem showing terms for directionality: adaxial (upper leaf surface), abaxial (lower leaf surface), proximal (nearer the stem) and distal (further from the stem). Credit: E.J. Hermsen (DEAL).
Compound leaves
The leaves of many plants are compound. A compound leaf is a single leaf that is divided into leaflets, or structures that themselves looks like individual leaves. Compound leaves can come in many forms, but the basic types are pinnate, palmate, and ternate (also known as trifoliate). The stalk of a leaflet (when present), is called a petiolule. The flat, planar portion of a leaflet may be called a blade or lamina in angiosperms (flowering plants), but different terminology is often applied to ferns (see below).
A pinnately compound leaf is a leaf that has a central axis, called the rachis, with lateral divisions (the term "pinnate" refers to a structure with feather-like organization). In the simplest case, a pinnately compound leaf is once-pinnate. In a once-pinnate leaf, leaflets are attached to the rachis. In a bipinnately compound leaf (twice-pinnate leaf), the rachis bears lateral axes, called rachillae (singular = rachilla), that themselves bear the leaflets. In a tripinnately compound leaf (thrice-pinnately compound), the rachillae bear secondary rachillae, that in turn bear the leaflets. (And so on.)
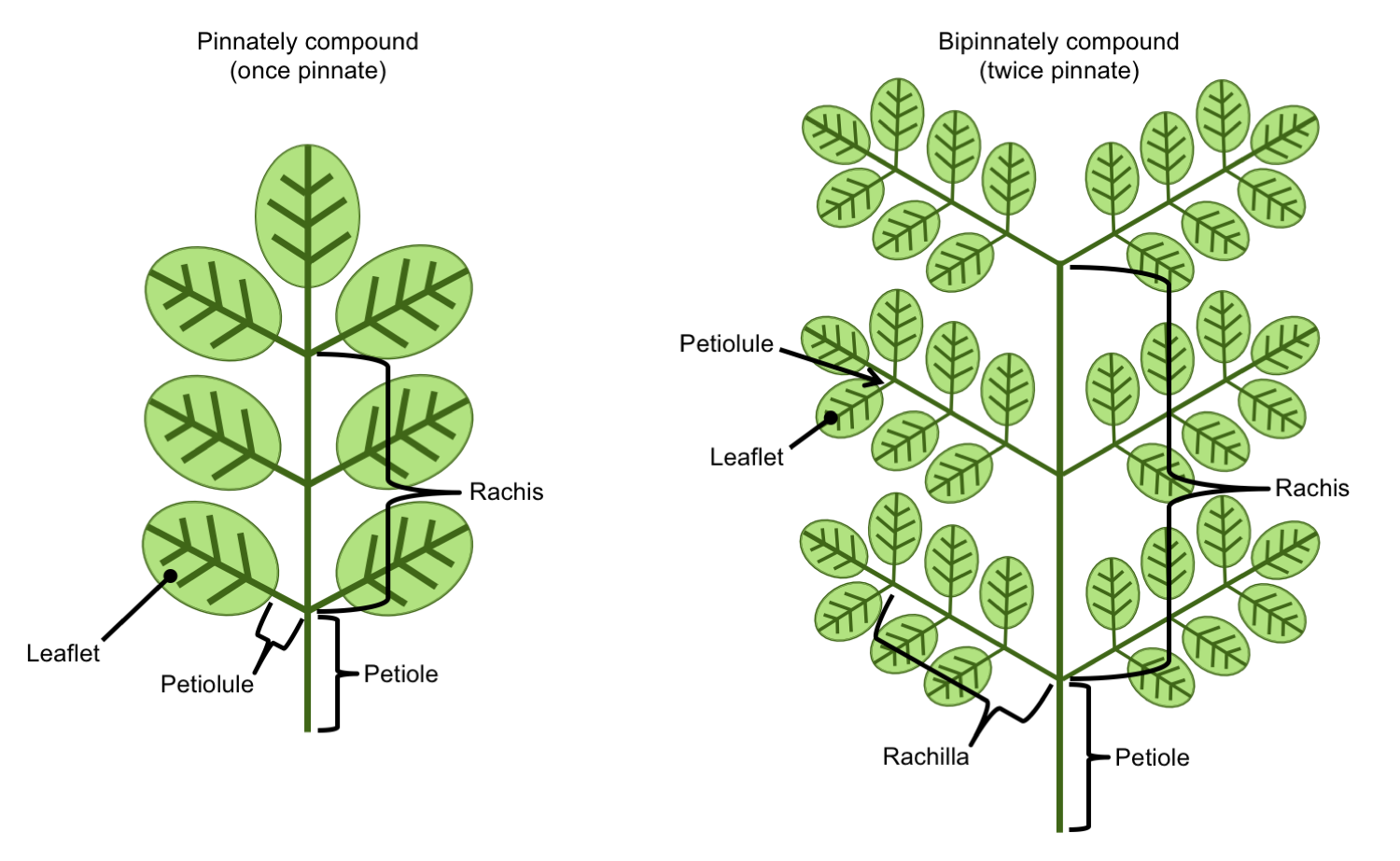
Pinnately compound leaves. Left: Once-pinnately compound leaf bearing petiolulate leaflets. Right: Twice-pinnately compound leaf bearing lateral rachillae (secondary axes); the rachillae bear the leaflets. Credit: E.J. Hermsen (DEAL).
In a palmately compound leaf, the leaflets are attached to the end of the petiole in a palmate arrangement (to remember the structure of a palmately compound leaf, think of the leaflets like fingers radiating from the palm of a hand). In a palmately compound leaf, there is no rachis. A ternately compound or trifoliate leaf is a compound leaf has three leaflets.
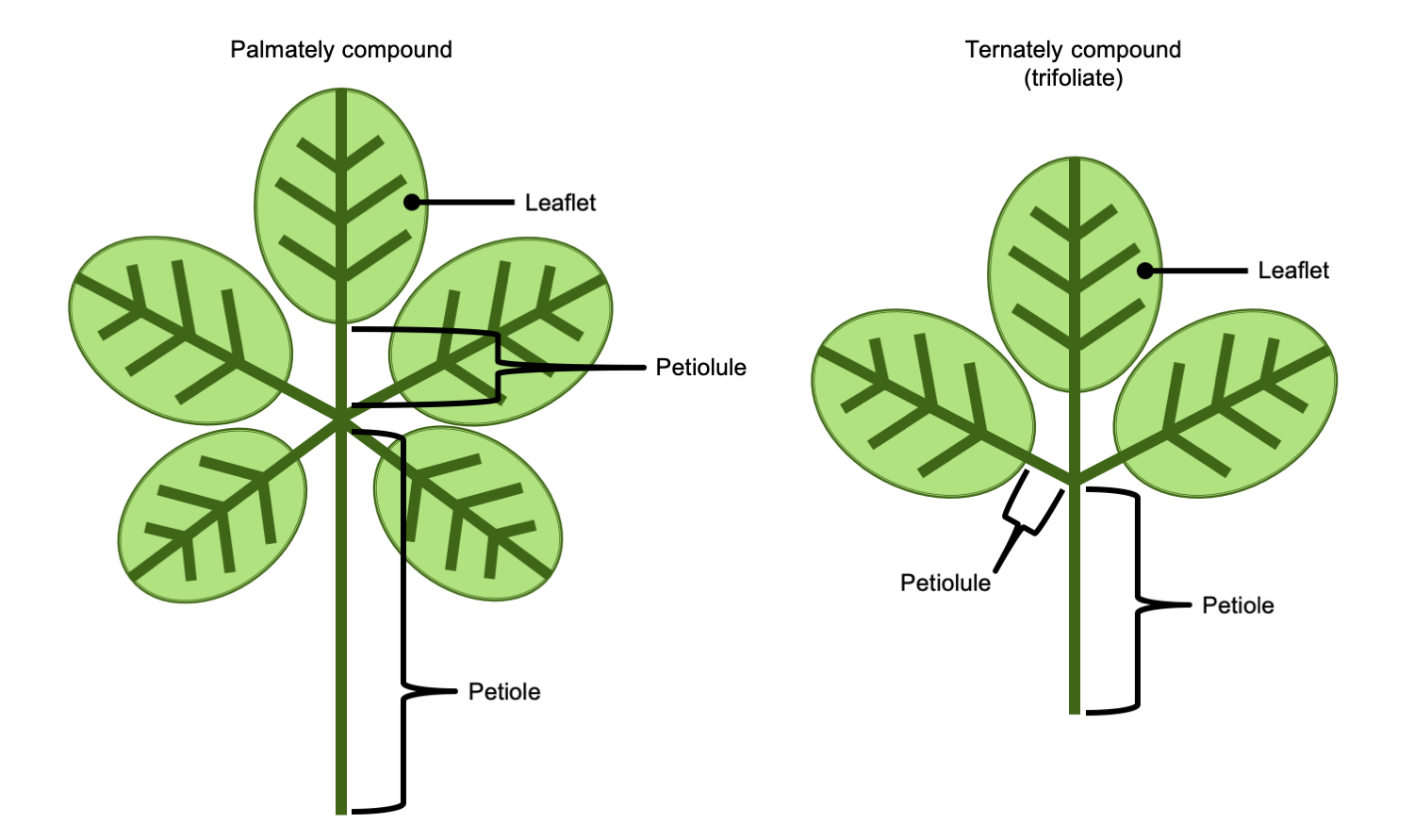
Palmately & ternately compound leaves. Left: Palmately compound leaf, with leaflets radiating from the end of the petiole; note that a palmately compound leaf has no rachis. Right: Ternately compound leaf (trifoliate leaf), a compound leaf with three leaflets; note that this ternately compound leaf does not have a rachis (thus, it can be called palmate-ternate). Credit: E.J. Hermsen (DEAL).
Alternative terminology: fern and fern-like leaves
The terms above can be considered "generic" terms for different regions of simple and compound leaves. They are also the terms commonly used to describe angiosperm (flowering plant) leaves. In other groups, particularly ferns and plants with fern-like leaves (like seed ferns and cycads), a different set of terms may be used. In other cases, terminologies may be mixed. The following account provides a brief overview of terms for ferns leaves, which can have highly complex structures.
A fern leaf is often called a frond, and the petiole of a frond may be called a stipe. The entire structure of the frond distal to the stipe (above the stipe in the diagrams below) is called the blade or the lamina, even if the frond is branching and divided into multiple leaflets.
The divisions of a fern frond are called segments. Some segments have special names:
- Pinnae (singular = pinna): The primary segments. Primary segments are the structures that are attached to the main rachis, or to the end of the stipe (petiole or stalk) if no rachis is present. The midvein or axis of a pinna is the costa. A midvein is a large, central vein that bisects a leaf or leaflet.
- Pinnules: The secondary segments. The secondary segments are the structures attached to the primary segments (pinnae). The midvein or axis of a pinnule is the costule.
- Higher-order segments: Higher-order segments are described by the degree of division that they represent. For example, segments attached to the pinnules (secondary segments) can be called tertiary segments.
The names for the leaflets differ depending on how many times the leaf is divided. For example, if a fern frond is once-pinnate, the leaflets are called pinnae. If a frond is bipinnate (twice-pinnate), the leaflets are the pinnules. If a leaf is tripinnate (thrice-pinnate) or even further divided, the leaflets may be called pinnules or ultimate segments (ultimate = last, referring to the highest order). Fern leaflets may be sessile (in other words, they may lack stalks). If a leaflet does have a stalk, the stalk can be called a petiolule. This term, however, is not often applied to ferns.
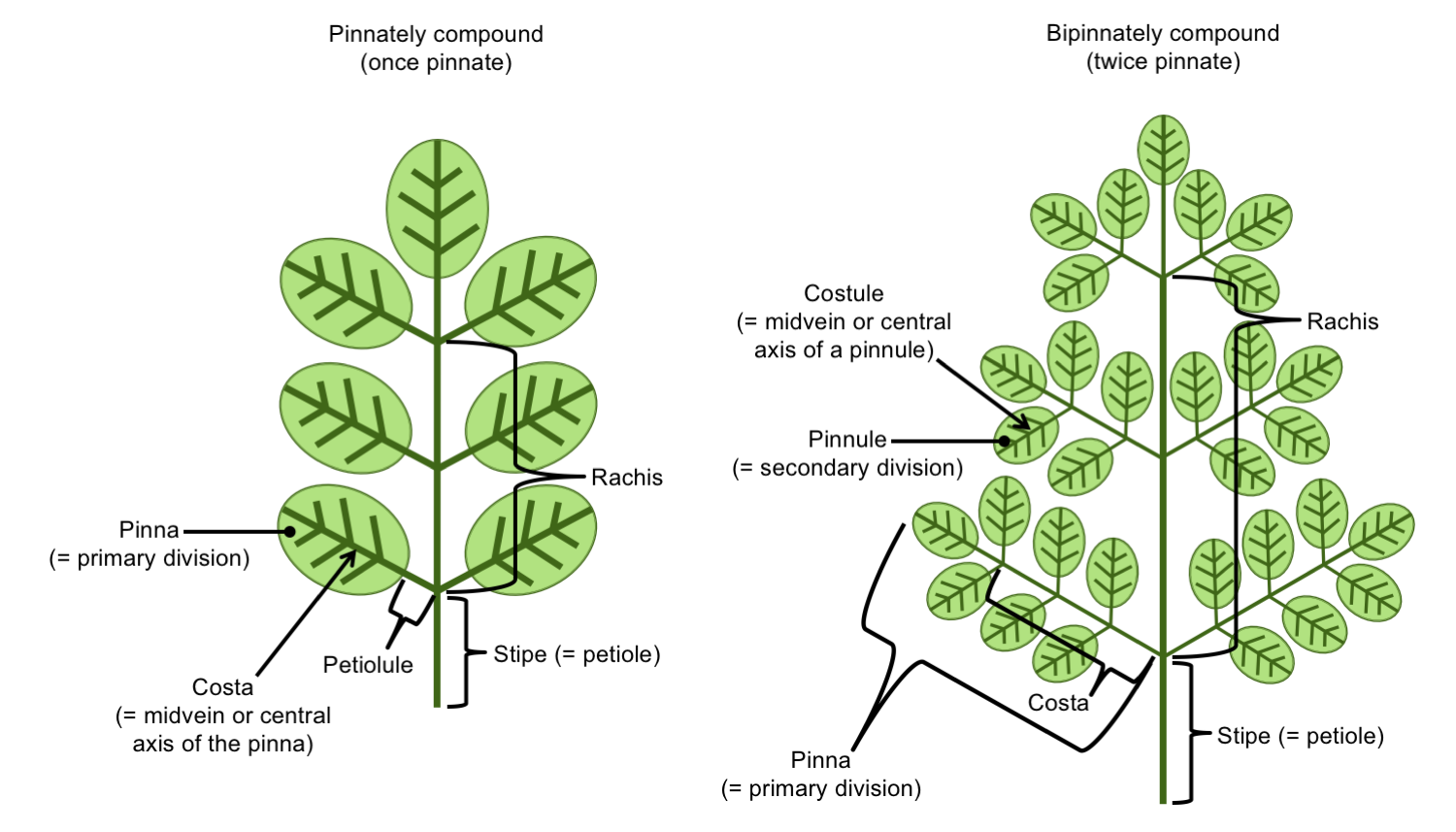
Terminology for pinnately compound fern fronds. Left: Once-pinnately compound frond, in which the pinnae (primary segments) are also the leaflets. Right: Twice-pinnately compound frond, where the pinnae are compound, and the pinnules (secondary segments) are the leaflets. Credit: Diagram by E.J. Hermsen (DEAL).
Leaf venation
A leaf contains one or more strands of vascular tissue, called veins, for transporting food and water. The leaf veins, especially the larger ones, are often easily observed on the surface of the leaf. (Shining a light through a leaf can help to make the venation stand out if it is difficult to see.) The pattern of venation in leaves varies depending on the group of plants and the size of the leaf. Venation patterns of leaves or leaflets can be important for identifying groups of plants in the fossil record. In flowering plants (angiosperms), complex terminologies have been devised to precisely describe leaf venation patterns.
When considering all vascular plants, the major categories of leaf venation can be generalized as follows:
- Single vein: A large, unbranched vein that bisects the lamina, called a midvein or midrib. Sometimes two unbranched veins will occur. This type of venation tends to be found in plants with simple, small and/or linear leaves like clubmosses (Lycopodium) and pines (Pinus).
- Dichotomizing venation: Veins fork one or more times, forming equal branches. This type of venation is characteristic of the maidenhair tree (Ginkgo) and occurs in other groups, like ferns.
- Parallel venation: The large veins in a leaf run parallel to one another and do not intersect, as in the leaves of grasses (Poaceae) and many other monocots (a large group of flowering plants), as well as some other groups like cordaites (an extinct group of plants related to conifers). Thin transverse (horizontal) veins may connect large veins.
- Reticulate venation: Veins anastomose (intersect or fuse) and form a net-like pattern. Veins may be similar in thickness, or some may be noticeably thicker than others. The enclosed areas formed by the veins are known areoles. This type of venation is found in some ferns, some seed ferns, and many flowering plants.
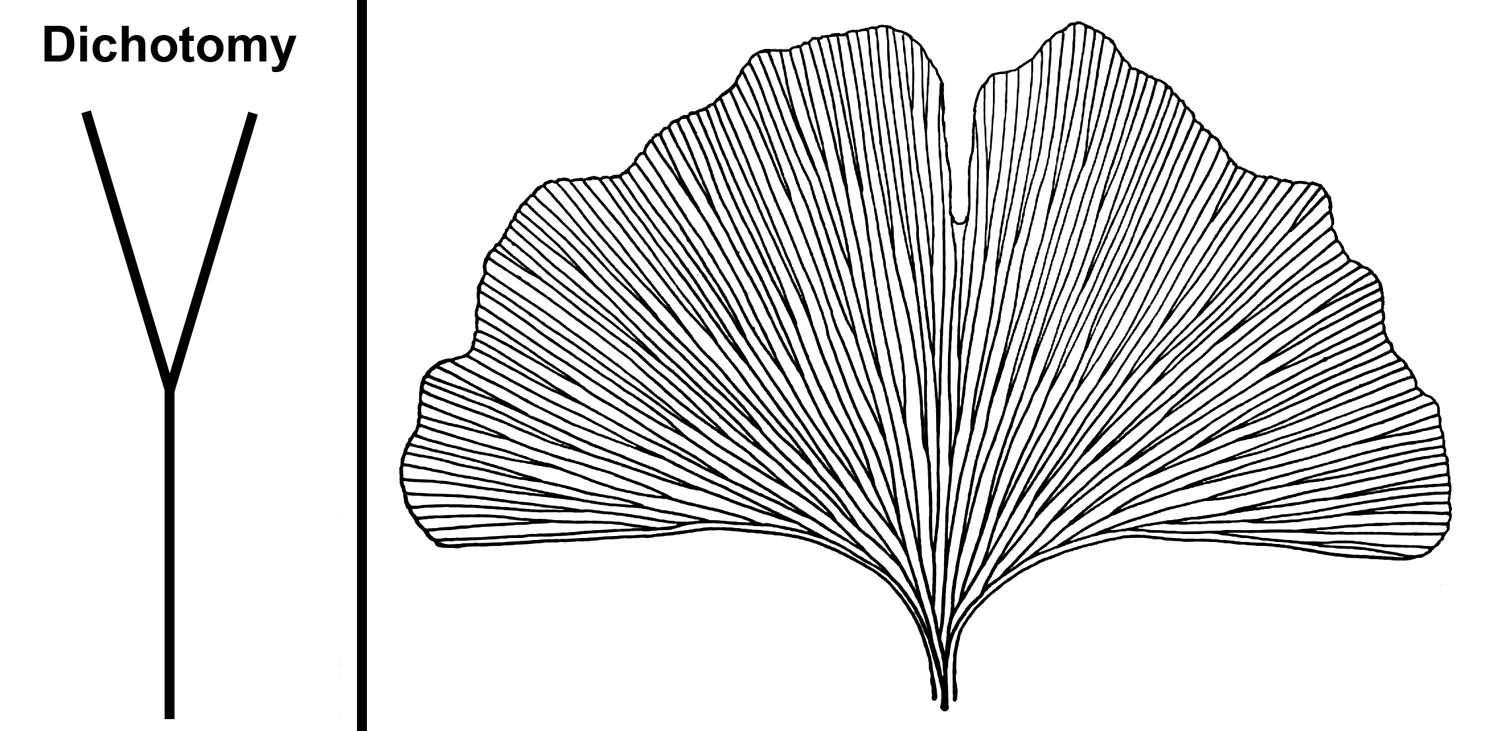
Dichotomizing venation. Left: Illustration of a dichotomy, where one vein divides to produce two equally sized veins. Right: Leaf of a ginkgo (also known as maidenhair tree, Ginkgo biloba) showing characteristic dichotomizing venation. Credits: Diagram of a dichotomy (E.J. Hermsen, DEAL); Drawing of a Ginkgo biloba leaf (fig. 465 in Coulter et al. 1910, via Internet Archive, presumed not in copyright, image modified from original).
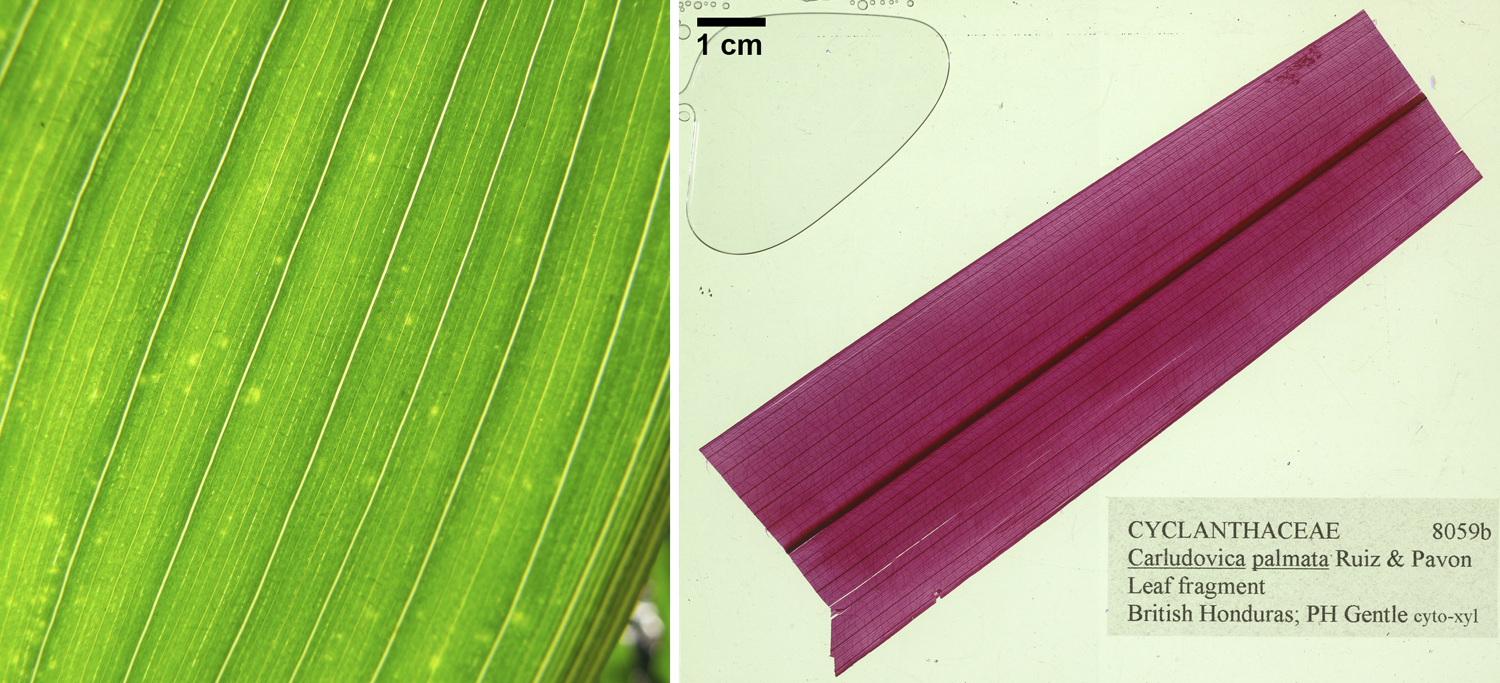
Parallel venation. Parallel venation or modified parallel venation is characteristic of the monocots, a large group of flowering plants; both of the leaves shown above are from monocots. Left: Close-up of a maize (corn, Zea mays) leaf, a type of grass (Poaceae). Right: Portion of a leaf of Panama hat plant (Carludovica palmata) showing a large central vein and thinner parallel veins to either side; this leaf fragment has been cleared and stained to emphasize the venation. Credits: Zea mays (corn) habit (Forest & Kim Starr, via Wikimedia Commons, CC BY 2.0); Carludovica palmata, YPM PB 167838 (S. Hu/Yale Peabody Museum, CC0 1.0/Public Domain Decidcation as indicated on the associated GBIF record). Images modified from originals.
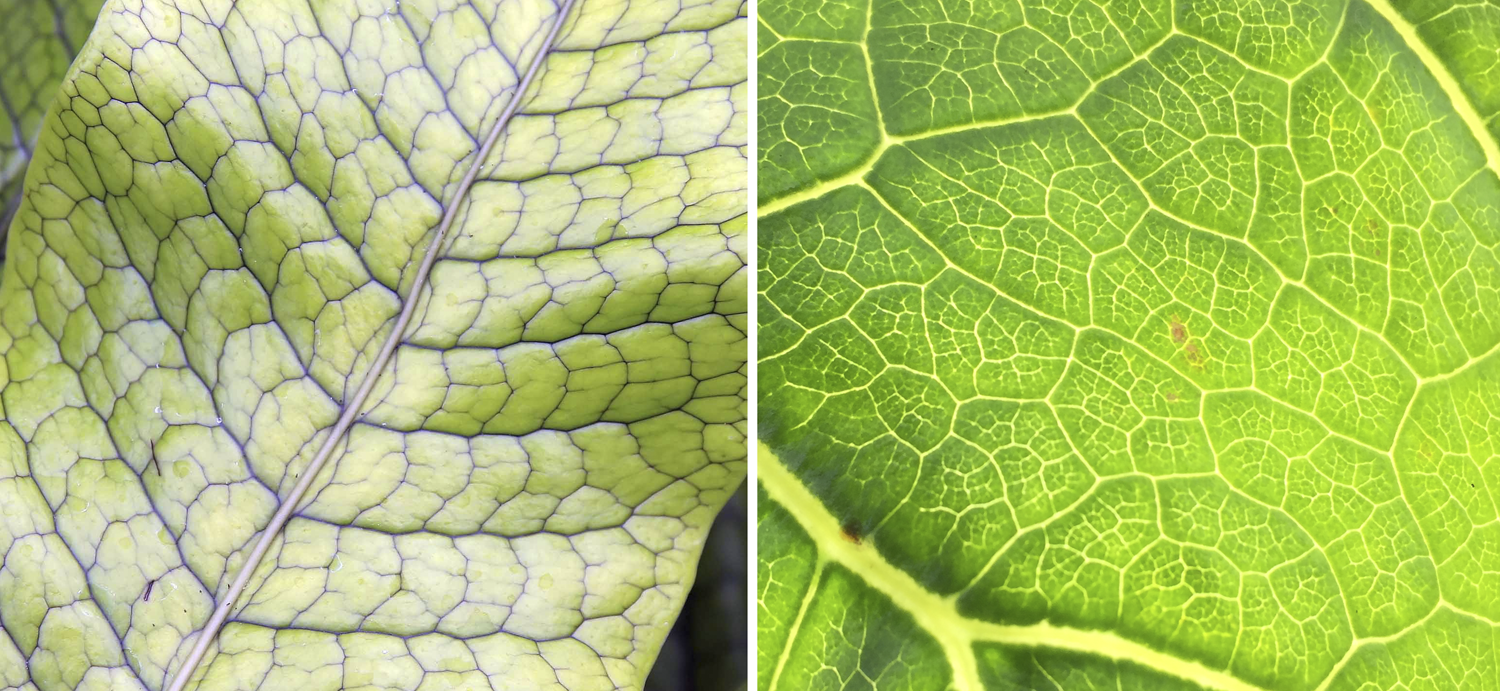
Reticulate venation. Left: Detail of a crocodile fern (Microsorum musifolium) leaf showing a midvein with reticulate venation to either side. Right: Detail of a cabbage tree (Dendroseris litroalis, a flowering plant) leaf showing complex reticulate pattern of minor veins that occur between the major veins. Credits: Microsorum musifolium (David J. Stang, via Wikimedia Commons, CC BY-SA 4.0); Dendroseris litoralis leaf venation detail (Flobbadob, via Wikimedia Commons, CC BY-SA 4.0). Images modified from originals.
Leaf anatomy
The internal structure of a leaf blade consists of layers of tissue. The surface of a leaf is made up of the epidermis, which is often only one cell thick. The outer surface of the epidermis is covered by a cuticle (fatty-waxy layer) to help prevent water loss. Stomata (also called stomates), or pores, in the epidermis allow for the exchange of gasses; each stoma has two guard cells that open and close the pore. (Note that the term "stoma" or "stomate" is often used to mean both a pore and its associated guard cells together.) The epidermis may also have trichomes (plant hairs), glands, etc. The upper epidermis covers the adaxial surface of the leaf, whereas the lower epidermis covers the abaxial surface of the leaf.
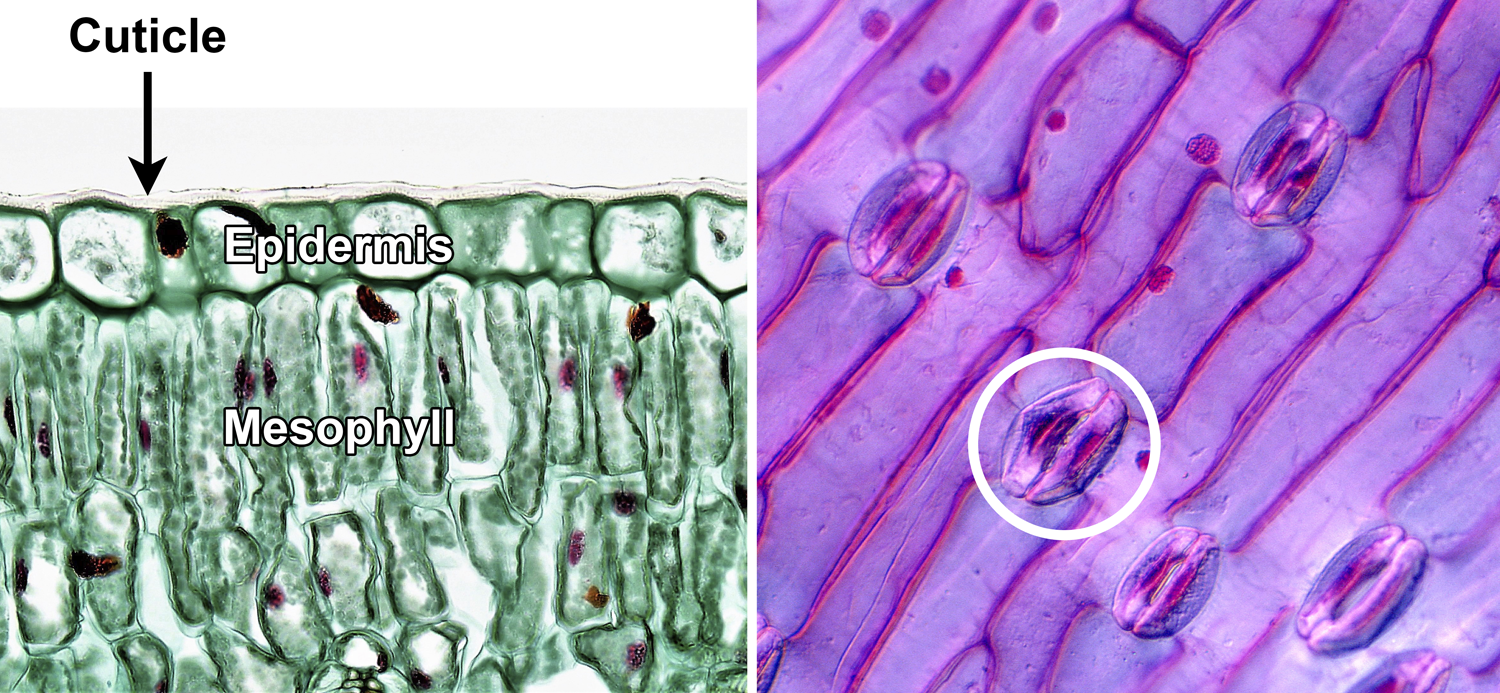
Leaf epidermis. Left: Cross section of a privet (Ligustrum) leaf, showing the adaxial (upper) epidermis, which is made up of a single layer of cells; the epidermis is covered to the cuticle. Note the mesophyll cells with nuclei (larger, red dots) and chloroplasts (smaller, green dots) under the epidermis. Right: Epidermis of a tulip (Tulipa) leaf, showing elongated epidermal cells and stomata; each stoma (pore) is flanked by two kidney-shaped guard cells (one stoma with guard cells is circled). Credits: Adaxial epidermis in Ligustrum (Fayette A. Reynolds M.S./Berkshire Community College Bioscience Image Library, via flickr, CC0 1.0/Public Domain Dedication); Tulip leaf epidermis (Josef Reischig, via Wikimedia Commons, CC BY-SA 3.0). Images modified from originals.
Filling in the space between the upper and lower epidermis is the mesophyll (Greek, mesos + phyllon = middle-leaf). The mesophyll is primarily made up of parenchyma cells. Parenchyma cells are thin-walled cells that play different roles in the plant body; in the leaf, one of their functions is to carry out photosynthesis. The leaf veins are embedded in the mesophyll. Veins may be surrounded by a distinct sheath of tissue known as a bundle sheath. Thick-walled cells (collenchyma cells or sometimes fibers) may be associated with larger leaf veins, helping to support the leaf.
The upper and lower regions of the mesophyll often look different. In a leaf with a thin, broad lamina, cells in the upper part of the mesophyll may be columnar (palisade parenchyma or palisade mesophyll), whereas cells in the lower part may be loosely organized (spongy parenchyma or spongy mesophyll). These two types of parenchyma occur because the cells of the upper mesophyll intercept sunlight and carry out photosynthesis, whereas most gas exchange takes place in the lower part of the mesophyll. Leaves with palisade parenchyma on top and spongy parenchyma below are known as dorsiventral or bifacial (two-faced), indicating that upper and lower sides are different.
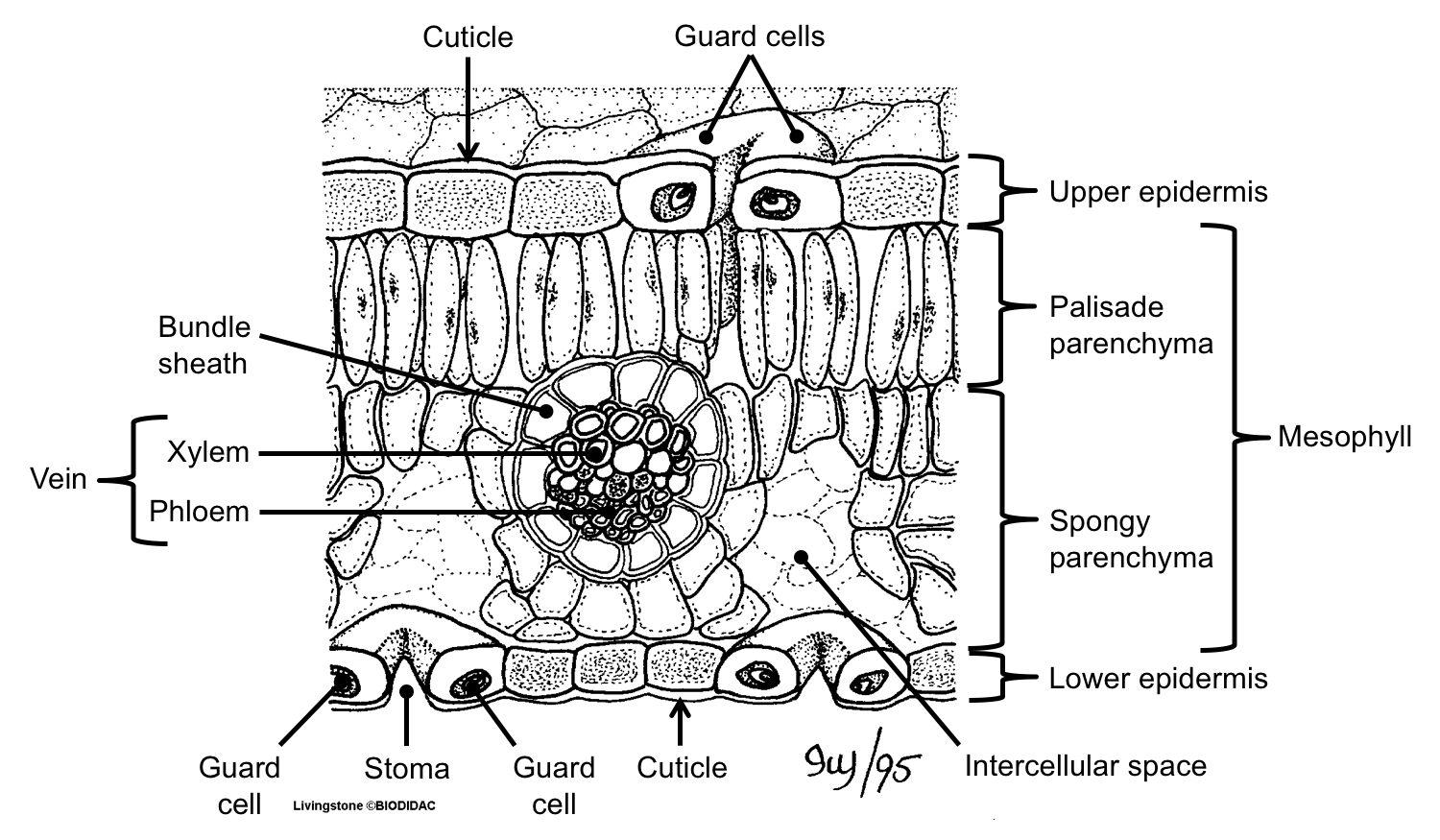
Cross-section of a dorsiventral (bifacial) leaf. The diagram above shows the blade (lamina) of a dorsiventral leaf in cross-section, with layered tissues. The uppermost and lowermost layers are the epidermis; the epidermis is covered by a cuticle and has stomata (pores) with guard cells that can open and close the pores. Filling the space inside the leaf is the mesophyll, with upper palisade parenchyma (columnar cells) and lower spongy parenchyma (irregularly-shaped cells). The vascular tissues make up the vein, which is surrounded by a bundle sheath. Credit: Cross-section of a leaf (Ivy Livingston, via BIODIDAC, CC BY-NC 4.0). Image modified from original.
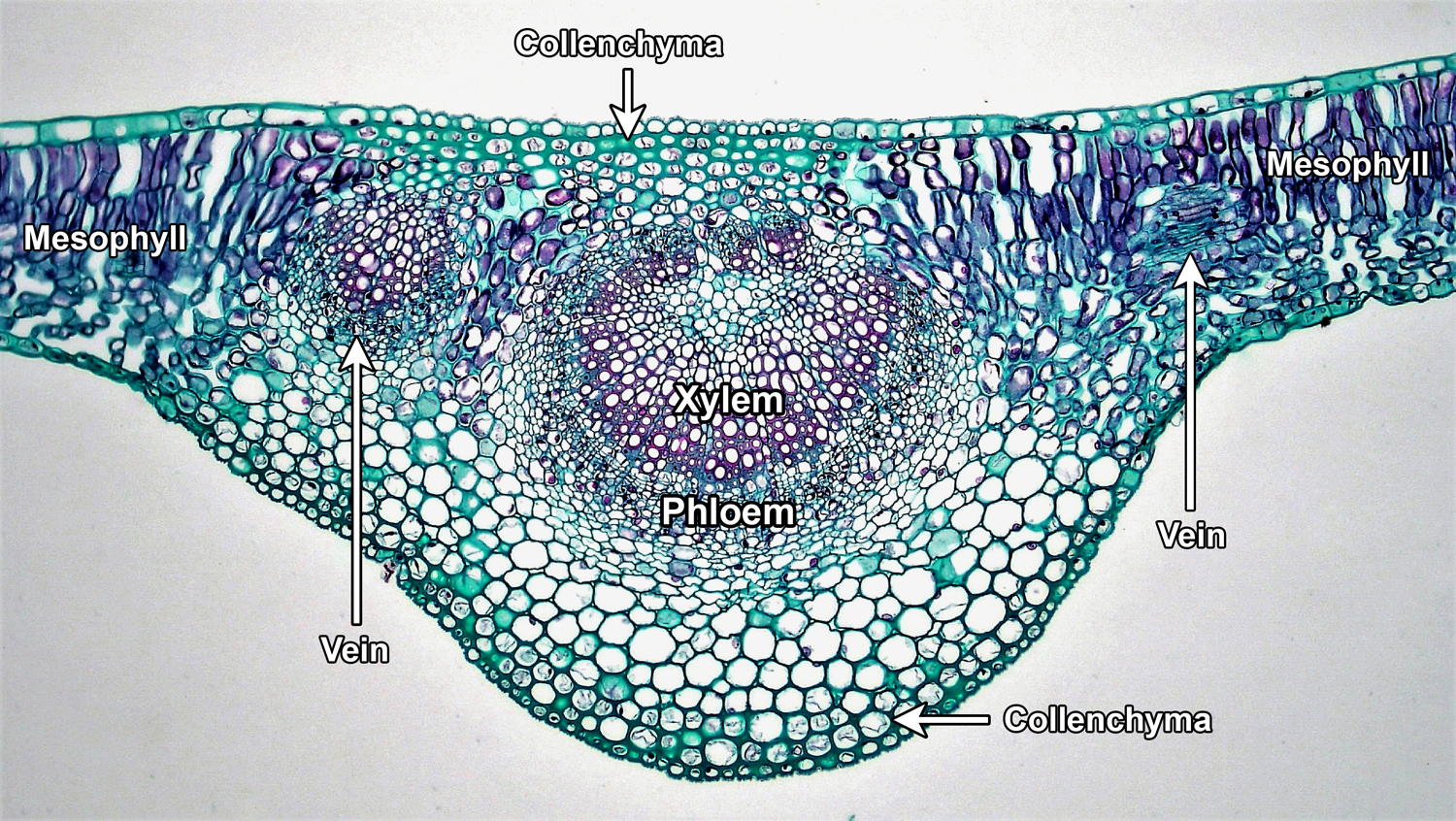
Cross-section of a leaf midvein. The section above was made through the midvein (large, central vein) of a lilac (Syringa) leaf. The midvein is in the center of the image, with xylem (water-conducting tissue) and phloem (food-conducting tissue) labelled. Surrounding the vascular tissues are thin-walled parenchyma cells and, under the upper and lower epidermis, thicker-walled collenchyma cells. Two smaller veins can be seen to the left and right of the midvein (the one on the right is sectioned obliquely). Palisade and spongy parenchyma cells making up the mesophyll can be seen at the periphery of the image. Credit: Mesophytic dicotyledonous leaf of Syringa, cross section (Fayette A. Reynolds M.S./Berkshire Community College Bioscience Image Library, via flickr, CC0 1.0/Public Domain Dedication). Image modified from original.
Some leaves may have palisade parenchyma on both upper and lower sides of the lamina, or the leaf mesophyll may look the same throughout the leaf. Lack of differentiation between the upper and lower parts of the mesophyll may be due to the orientation of the leaves (for example, both sides of the leaf intercept sunlight), their form, and other adaptations. Certain flowering plants, called C4 plants, often have mesophyll that is concentrically arranged around the vascular bundles. This arrangement is known as kranz anatomy (German Kranz = wreath) and is related to the type of photosynthesis found in these plants, known as C4 photosynthesis. Most C4 plants are grasses.
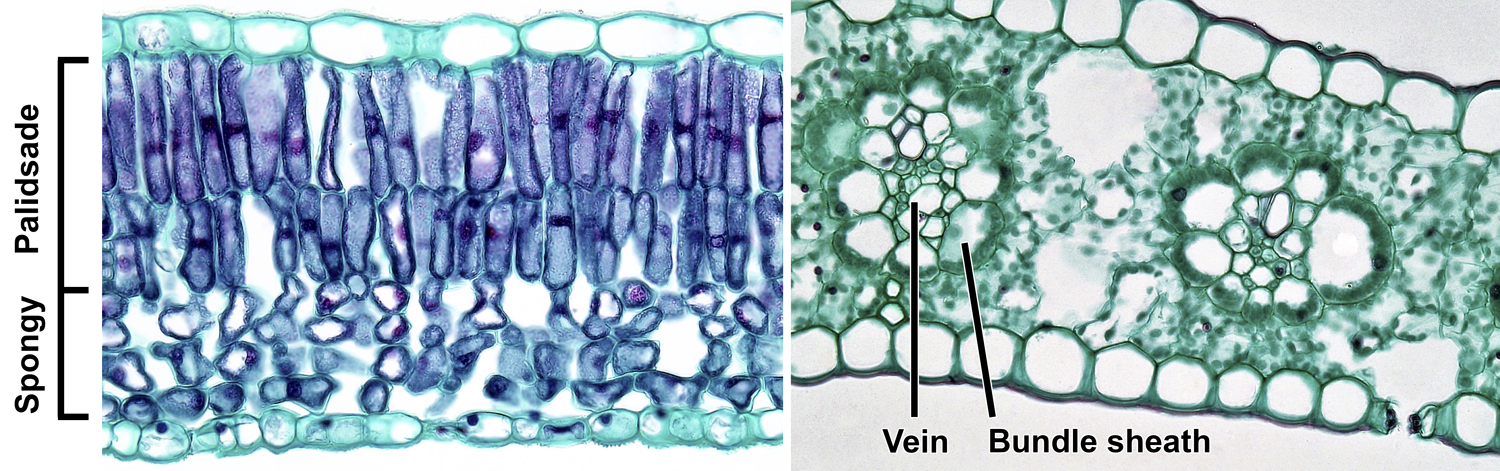
Mesophyll of angiosperm (flowering plant) leaves. Left: Cross section of the lamina of a lilac (Syringa) leaf, showing bifacial (dorsiventral) structure; the upper palisade parenchyma consists of two layers of cells, and the lower spongy parenchyma has irregularly-shaped cells with large spaces between them. No veins are visible. Right: Cross section of the blade of a maize (Zea mays) leaf, showing kranz anatomy; note two veins surrounded by conspicuous bundle sheaths, which are in turn surrounded by mesophyll cells (the green dots in the cells are chloroplasts). A stoma can be seen in the abaxial (lower) epidermis, to the right. Credits: Mesophyll arrangement in Syringa leaf and Mesophyll arrangement in Zea leaf (Fayette A. Reynolds M.S./Berkshire Community College Bioscience Image Library, via flickr, CC0 1.0/Public Domain Dedication). Images modified from originals.
Other variations are found small, narrow, or highly simplified leaves. In the scale-like leaves of a clubmoss (Lycopodium), the mesophyll looks similar throughout the leaf. The needle-like leaves of pine (Pinus) are more complex. The mesophyll, which is not differentiated into palisade and spongy layers, surrounds a central region that includes (from outside to inside), a layer of endodermis, transfusion tissue, and one or two vascular bundles (veins). Transfusion tissue is common in gymnosperm leaves. It is made up of parenchyma cells and tracheids (a type of water-conducting cell) and is associated with vascular bundles.
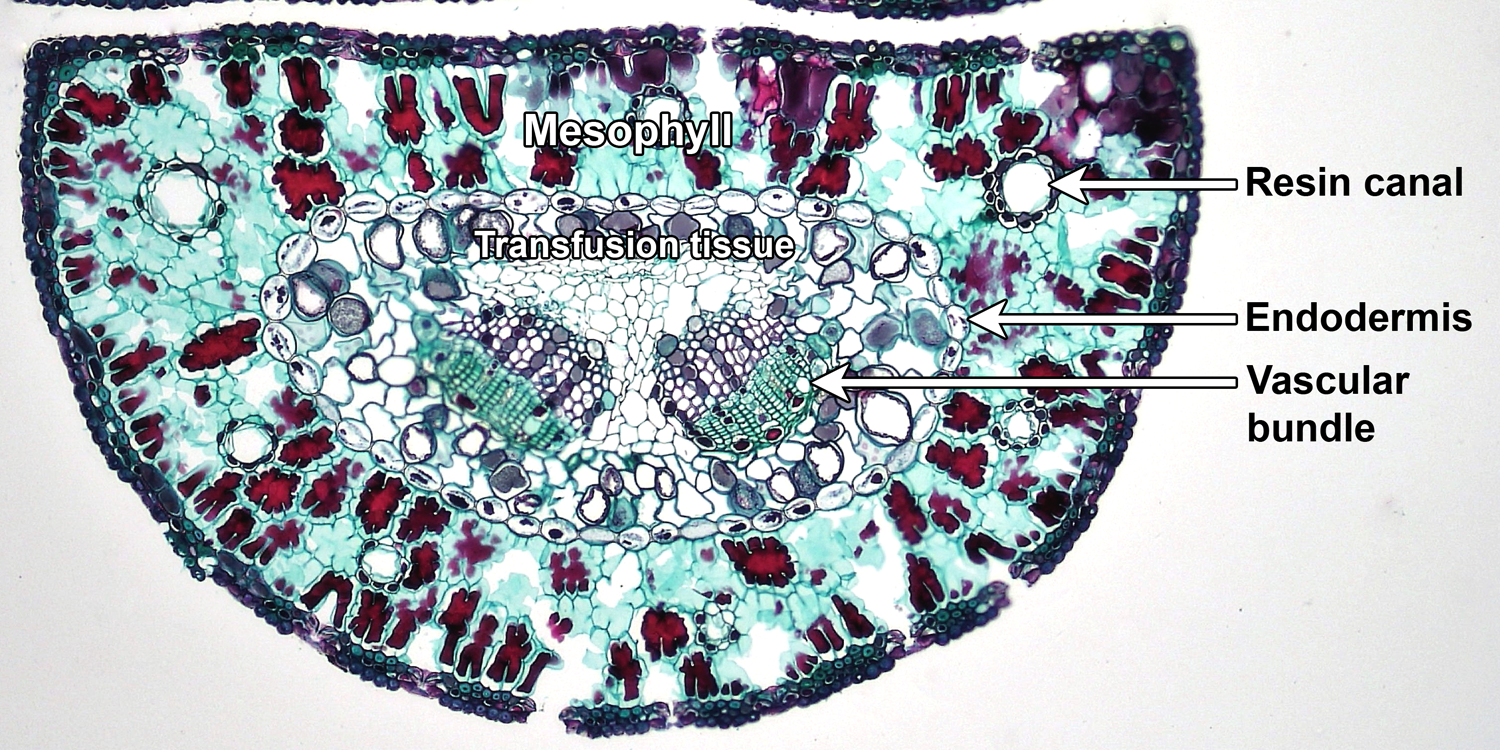
Anatomy of a pine (Pinus) leaf. Cross section of a pine leaf, showing the uniform mesophyll with large resin canals surrounding a central region bounded by the endodermis; interior to the endodermis, two vascular bundles (veins) are embedded in transfusion tissue. Credit: Two needle Pinus 100x (Fayette A. Reynolds M.S./Berkshire Community College Bioscience Image Library, via flickr, CC0 1.0/Public Domain Dedication). Image modified from original.
Leaf anatomy may have modifications related to the environment that a particular plant inhabits. Plants adapted to aquatic environments may have large air spaces in the mesophyll of their leaves. This parenchyma with air spaces is known as aerenchyma (Greek aēr = air). Aerenchyma provides buoyancy and can help gasses circulate within tissues. Conversely, leaves of plants living in arid environments show modifications to prevent water loss. These may include such features as thick cuticles, a multiple epidermis (multi-layered epidermis), and sunken stomata.
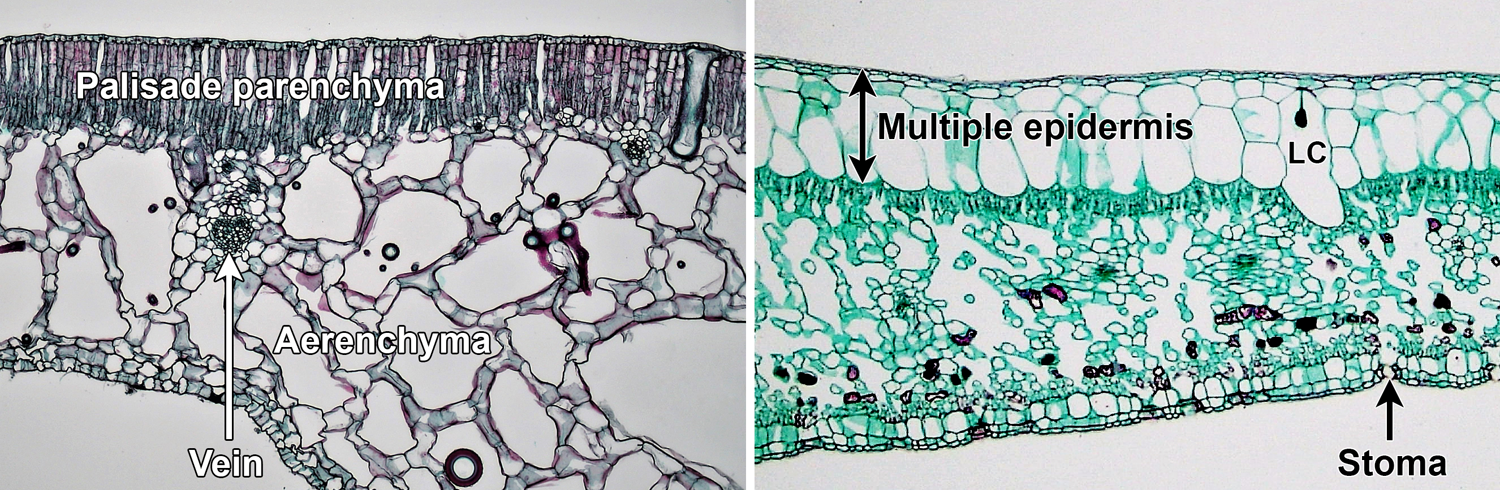
Leaf adaptations for aquatic and xeric environments. Left: Leaf of water lily (Nymphaea), an aquatic plant, showing aerenchyma (in this case, spongy parenchyma with very large intercellular spaces) beneath the palisade parenchyma. Right: Leaf of fig (Ficus), a plant of arid environments, with a multi-layered epidermis; note that the epidermis is better developed on the upper side of the leaf, and the stomata are on the lower surface. The cell labeled LC is a lithocyst (a cell with calcium carbonate body attached to its wall). Credits: Mesophyll arrangement in Nymphaea 100x and xerophytic leaf of Ficus 40x (Fayette A. Reynolds, M.S./Berkshire Community College Bioscience Image Library, via flickr, CC0 1.0/Public Domain Dedication). Images modified from originals.
Phyllotaxy
Leaves are arranged in a regular sequence, known as phyllotaxy (or phyllotaxis) around the stem. There are many different terms that can be used to described phyllotaxy. Some terms indicate how many leaves occur per node. A node is a region on a stem where one or more leaves are attached. The basic terms describing the number of leaves per node are as follows:
- Alternate: Leaves occur one at a time on the stem.
- Opposite: Leaves occur in pairs.
- Whorled: Leaves occur in groups of three or more around the stem.
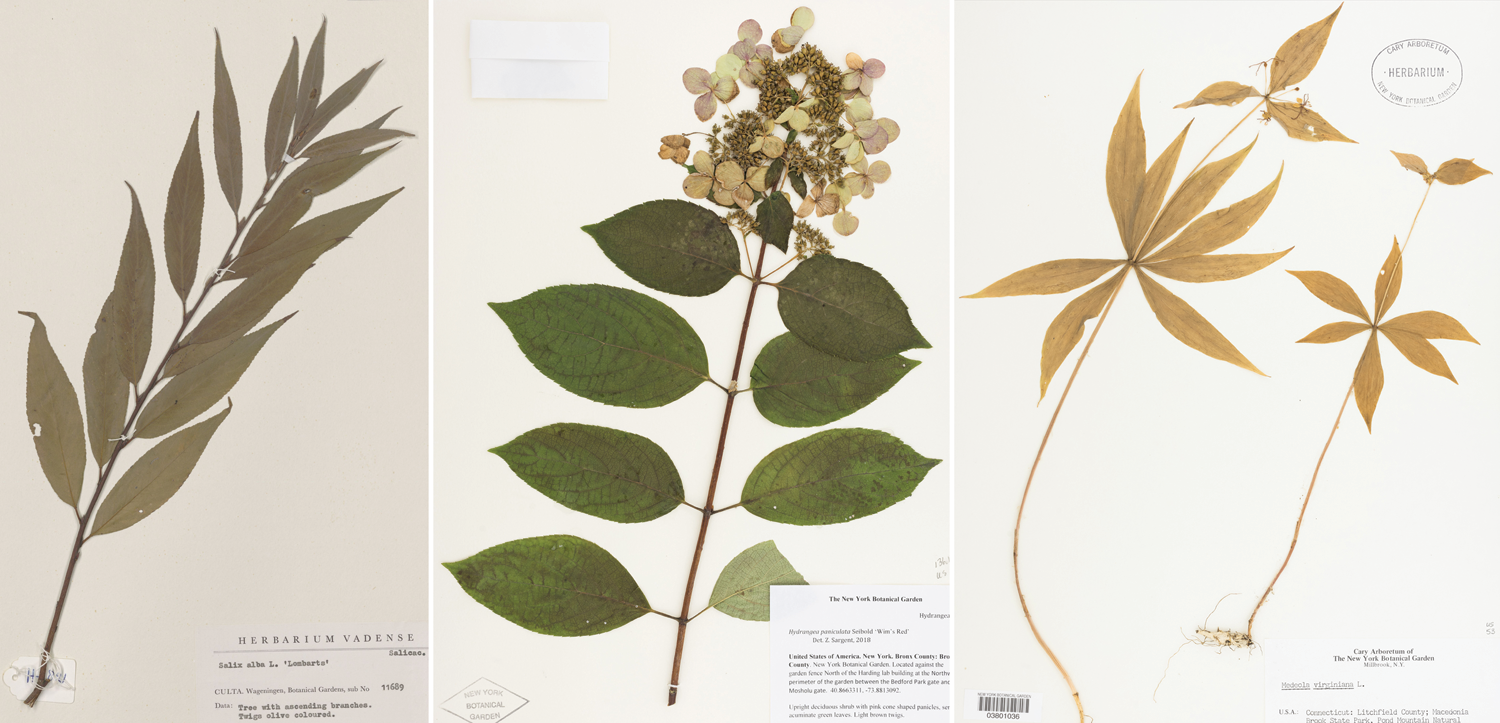
Phyllotaxy. These three herbarium specimens (pressed, dried plants or plant parts) show the three basic types of phyllotaxy. Left: White willow (Salix alba), alternate leaves. Center: Panicle hydrangea (Hydrangea paniculata), opposite leaves. Right: Cucumber-root (Medeola virginiana), whorled leaves. Credits: Salix alba, WAG.1211140 (Naturalis Biodiversity Center, via GBIF, CC0 1.0/Public Domain Dedication); Hydrangea paniculata, NY 03350084, Zoe Sargent 2 (The New York Botanical Garden, via GBIF, CC BY 4.0); Medeola virginiana, NY 03801036, G. Feldman 50 (The New York Botanical Garden, via GBIF, CC BY 4.0).
These terms can be combined with terms used to describe the vertical alignment or arrangement of leaves on a stem for more precision. Examples of such terms include:
- Distichous: Leaves are aligned in two vertical rows along the length of the stem; also known as two-ranked. This term can be used for plants that have alternate or opposite leaves.
- Decussate: A common arrangement of opposite leaves, where each leaf pair is perpendicular to the pair above and below it. Thus, leaves form four vertical rows on the stem.
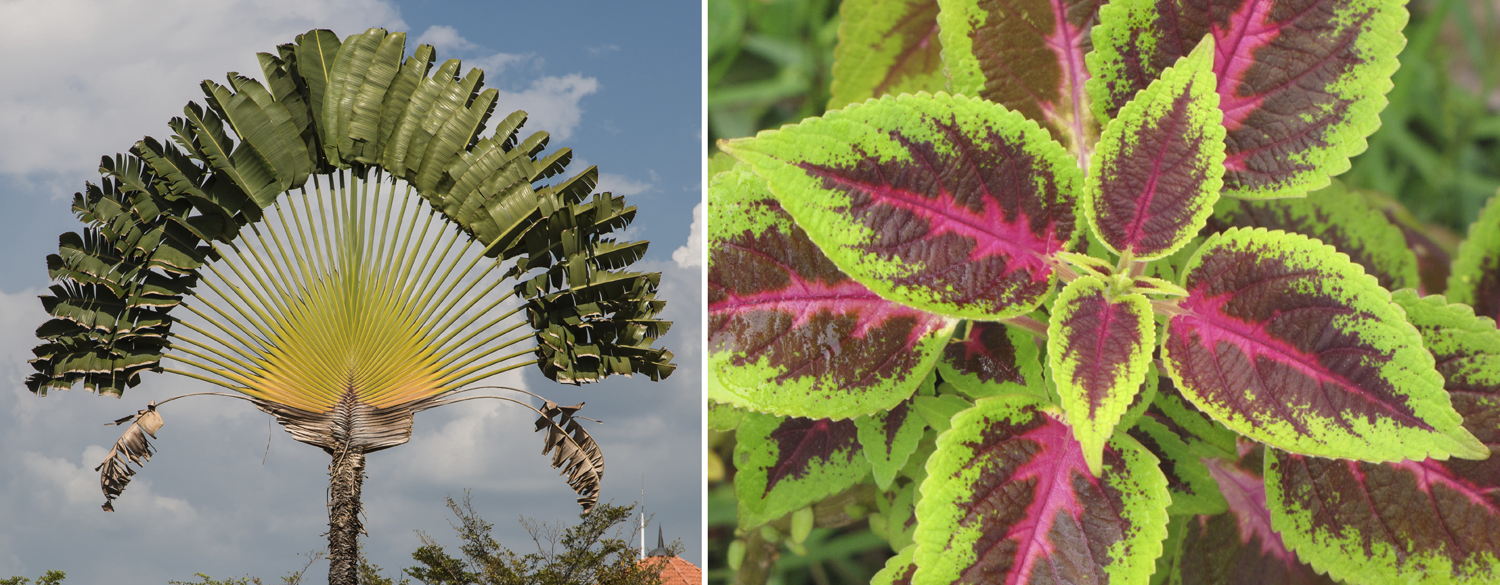
Distichous and decussate leaf arrangements. Left: Traveler's tree (Ravenala madagascariensis) showing a distichous leaf arrangement. Right: Coleus (Plectranthus scutellarioides) showing a decussate leaf arrangement. Credits: Penang Malaysia Ravenala madagascariensis (CEphoto, Uwe Aranas, via Wikimedia Commons, CC BY-SA 3.0); Plectranthus scutellarioides (Forest & Kim Starr, via Wikimedia Commons, CC BY 3.0 US). Images modified from originals.
A few special cases are worth noting because they are important in describing the phyllotaxis of gymnosperms and lycophytes. The term spiral or helical may be used where leaves are closely spaced and form a tight helix on the stem. Note that spiral can also be used to refer to a type of alternate phyllotaxis where the leaves form a loose helix around the stem.
Spirally arranged leaves often occur on lycophytes and in cycads. In arborescent (tree-like) lycophytes (the extinct order Lepidodendrales), cycads, and cycadeoids (an extinct group of superficially cycad-like plants), the helical arrangement of the leaves can often be observed by tracing the arrangement of leaf scars left on the stems after leaves are shed.
Spirally or helically arranged leaves. The model above shows a portion of the stem of Lepidodendron, an extinct, tree-sized lycophyte. The diamond-shaped structures on the surface of the stem are leaf cushions that mark the former positions of the leaves. Note the spiral or helical arrangement of the leaf cushions, which indicate the phyllotaxis of the leaves. Specimen from the Carboniferous of Indiana, U.S.A.; approximate length = 20 cm. Credit: Lepidodendron stem specimen from the Cornell University Paleobotanical Collection (CUPC), no number (Model by Emily Hauf/Digital Atlas of Ancient Life, via Sketchfab, CC BY-SA 4.0).
A fascicle is a group of leaves in a bunch or cluster. Structurally, leaf fascicles are leaves borne close together on short shoots or spur shoots (also sometimes called dwarf shoots, particularly in pines). As the name implies, a short shoot is a tiny lateral branch.
Pines (Pinus) have leaves in fascicles. In pines, each fascicle has a set number of needle leaves; counting the needles per fascicle can aid in identification. In ginkgo (Ginkgo biloba), the fascicled leaves on short shoots are close together and spirally arranged, without a set number of leaves per short shoot. Alternately arranged leaves occur on long shoots. Long shoots are shoots with large gaps, or internodes, between consecutive leaves or consecutive short shoots.
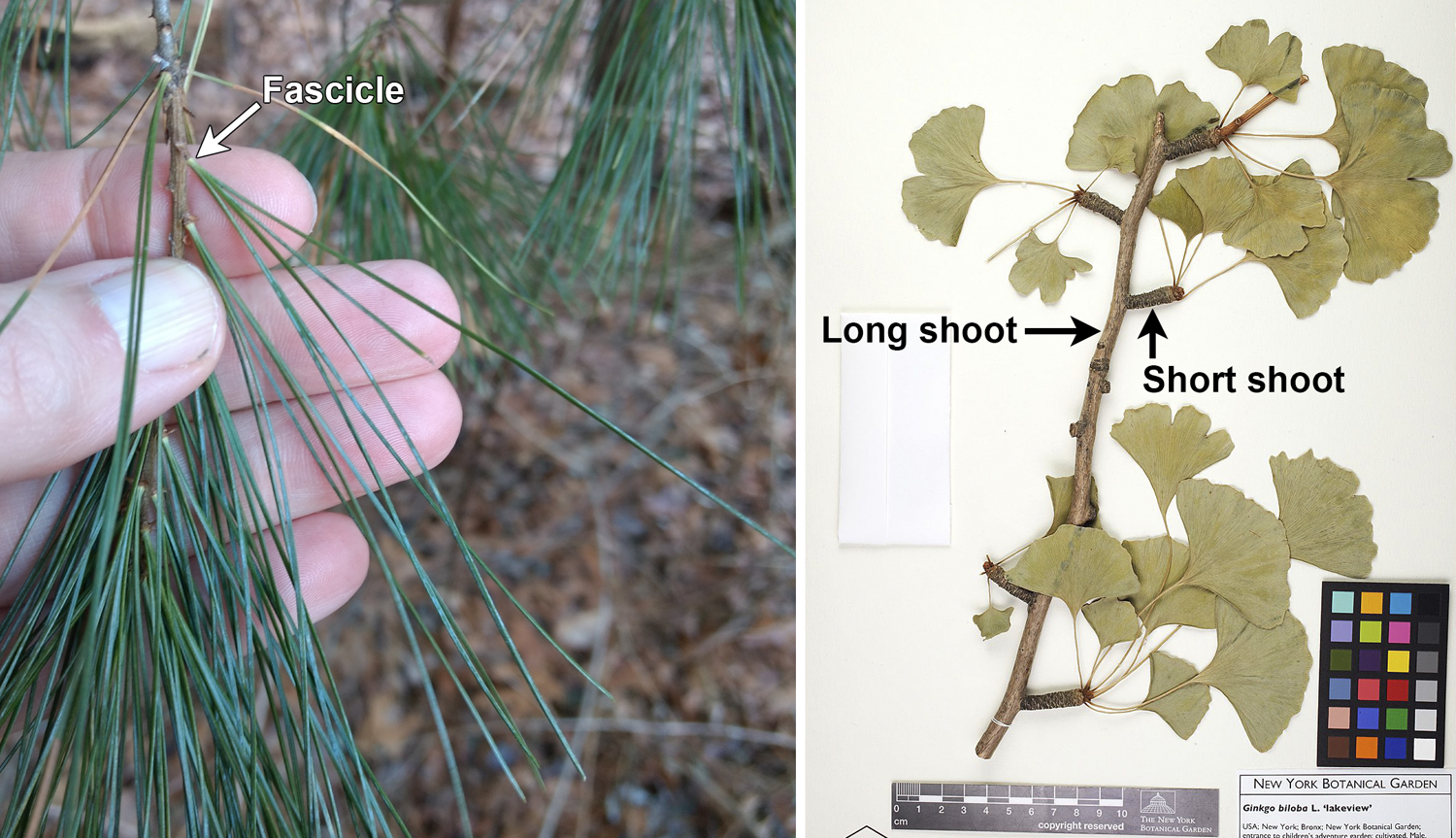
Fascicled leaves. Left: Eastern white pine (Pinus strobus), a pine with five needles per fascicle. Right: A herbarium sheet with a portion of a ginkgo (Ginkgo biloba) branch, showing a long shoot bearing several short shoots; note the clusters of leaves at the ends of the short shoots. Credits: Eastern white pine/Pinus strobus, Photo 29770637 (Mark Apgar, via iNaturalist, CC BY 4.0); Ginkgo biloba, Damon P. Little and Eric D. Brenner No. 917, NYBG 00759239 (New York Botanical Garden, via GBIF, CC BY 4.0).
The origins of leaves
Two basic types of leaves occur in vascular plants, microphylls (Greek mikros + phyllon = small leaf) and megaphylls (Greek megas + phyllon = large leaf). These terms are used to distinguish leaf types that differ in their evolutionary origins and, often, their structural attributes. Some scientists have criticized these terms as confusing because small, simple megaphylls look similar to microphylls. Furthermore, the terms are sometimes used in a purely descriptive way with no evolutionary implications. Thus, it has been proposed that the two major leaf types in vascular plants instead be called lycophylls (= microphylls) and euphylls (= megaphylls).
Microphylls are the leaves of lycophytes. These leaves lack a stalk (petiole or stipe), are simple in form (often scale-like or strap-shaped), and typically have a single vein that does not branch. Because lycophytes have stems with protosteles, microphylls are not associated with leaf gaps (a leaf gap is a gap in the stem vascular tissue that occurs above the point at which a leaf trace departs).
Megaphylls are the leaves of euphyllophytes. Megaphylls are highly diverse in form and may have complex venation; however, in some taxa like horsetails (Equisetum) and many conifers (like pines, Pinus), megaphylls are small with very simple venation and resemble microphylls. Typically, megaphylls are associated with leaf gaps because they are found in plants that have stems with siphonosteles (vascular tissue forms an open cylinder around a pith) or eusteles (vascular tissue occurs in separate bundles).
Microphylls have evolved once in vascular plants and are a synapomorphy for the lycophytes. Megaphylls have evolved more than once in euphyllophytes, so they are not a true synapomorphy for the whole group. (For this reason, it has been proposed that the "megaphyll" or "euphyll" concept be abandoned altogether—see here). However, overtopping, one of the early steps in the hypothesized evolution of the megaphyll (see below), is considered a synapomorphy for euphyllophytes.
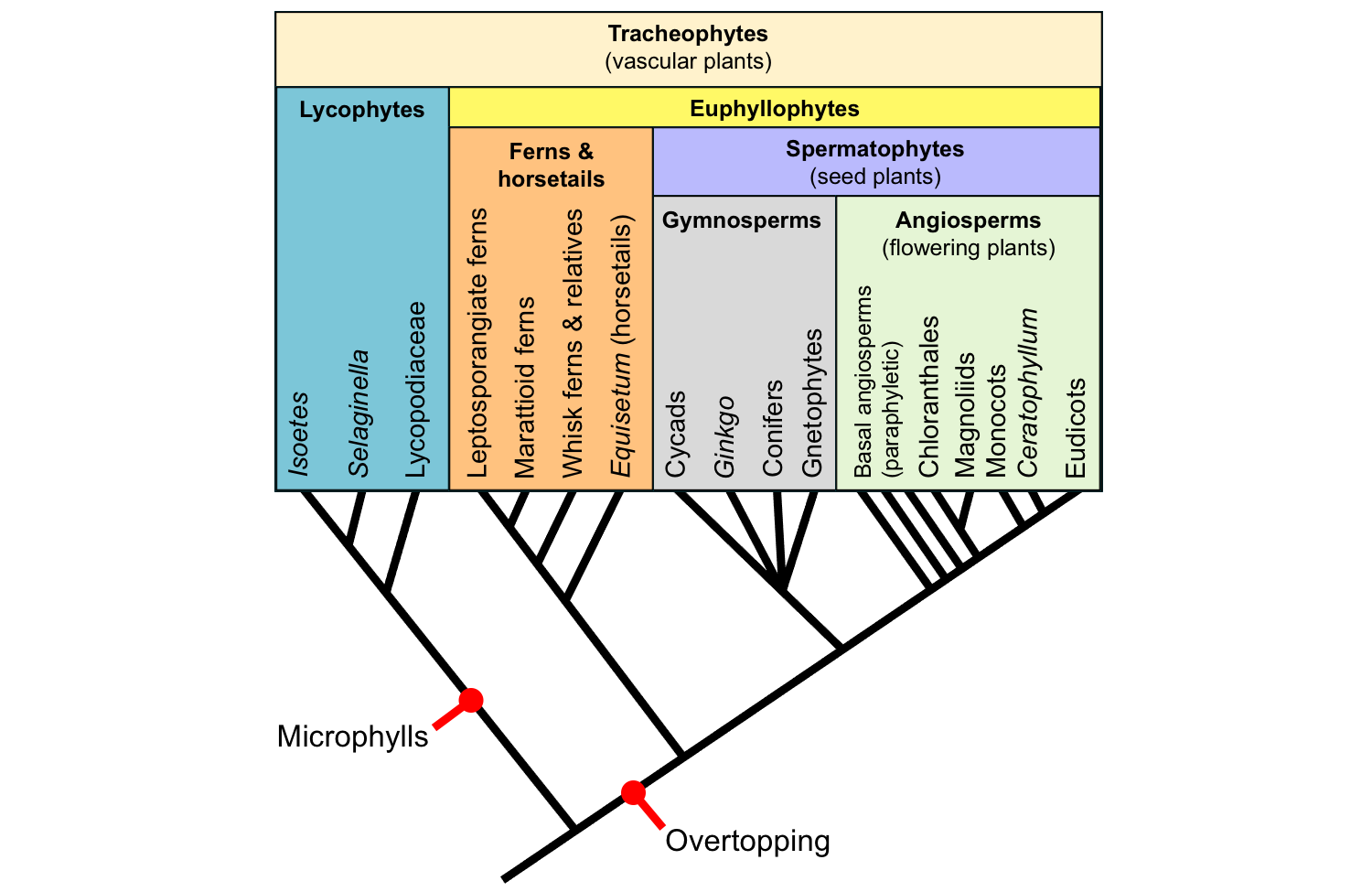
Origins of leaves. The tree of living tracheophyte (vascular plant) relationships above shows key steps in the origins of leaves. Microphylls are a synapomorphy for the lycophytes. Overtopping, one of the steps in the hypothesized origin of megaphylls, is a synapomorphy for euphyllophytes. Credit: E.J. Hermsen (DEAL).
Origin of microphylls (lycophylls)
The theory that explains the origin of microphyll is the enation theory. Under this theory, microphylls evolved from structures known as enations, which are flap-like outgrowths of the stem that lack vascular tissue. The steps in this theory are as follows:
- Plants have naked aerial axes (stems) with no outgrowths.
- Origin of enations: Flap-like outgrowths develop on aerial axes (stems).
- Leaf traces: Leaf traces develop, but do not enter the enation.
- Leaf vascularization: The enations become vascularized and can be called leaves.
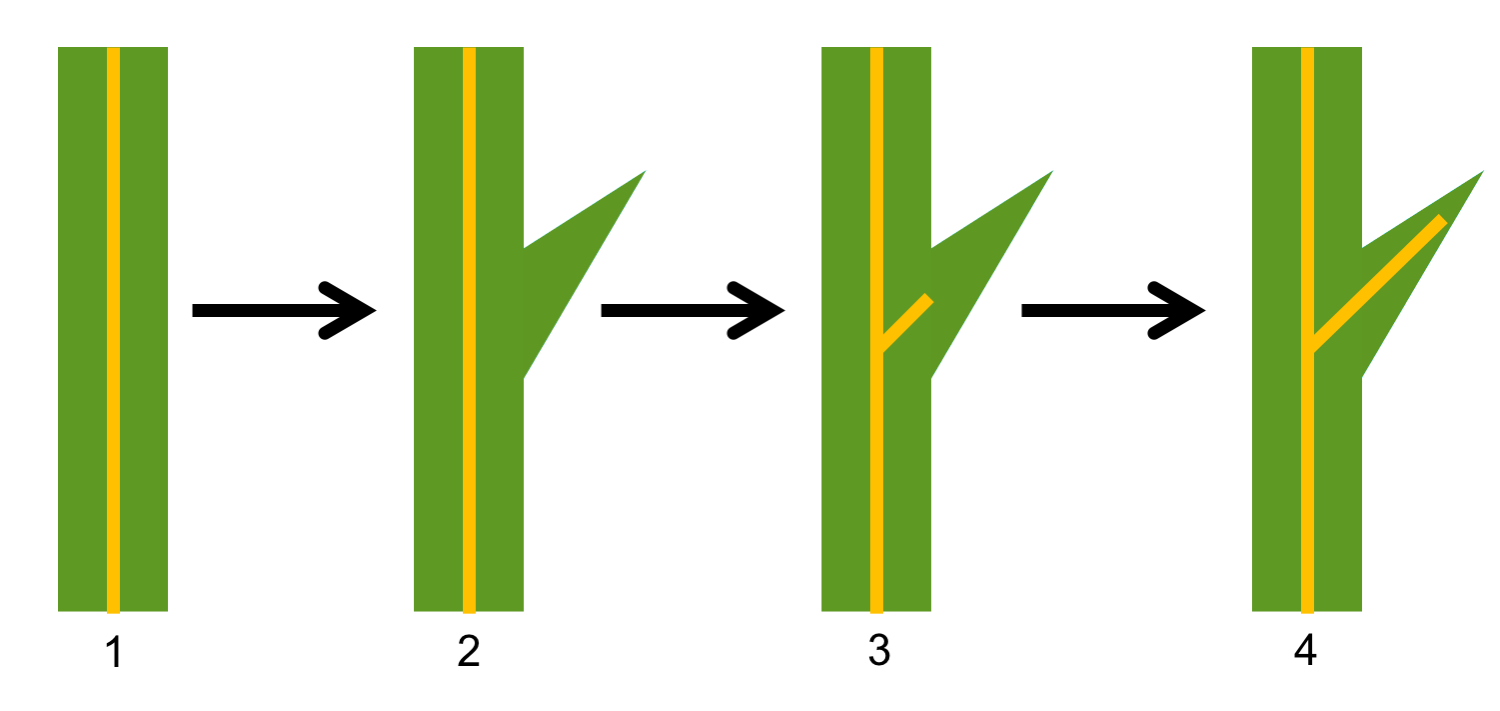
Enation theory for origin of microphylls (lycophylls). The steps in the enation theory, which is a theory for the origin of lycophyte leaves, is as follows: 1. Leafless axis (the vascular tissue is represented by the vertical yellow stripe). 2. Axis with an enation; note that no vascular tissue diverges toward the enation. 3. A leaf trace diverges toward the enation, but does not enter it. 4. Vascular tissue enters the enation, which is now a leaf. Credit: Diagram by E.J. Hermsen (DEAL). After fig. 3-9 in Gifford & Foster (1974), among other sources.
Origin of megaphylls (euphylls)
The classical explanation for the origin of megaphylls is the telome theory. This theory proposes that megaphylls evolved from branching systems. The steps in the evolution of the megaphyll under the telome theory are as follows:
- Plants have axes (stems) with equal dichotomous branching.
- Overtopping: Branching becomes unequal so that plants have a dominant stem bearing branching systems.
- Planation: The branching systems became planar (flattened).
- Webbing: A lamina (flat sheet of tissue) develops between branches to form a leaf.
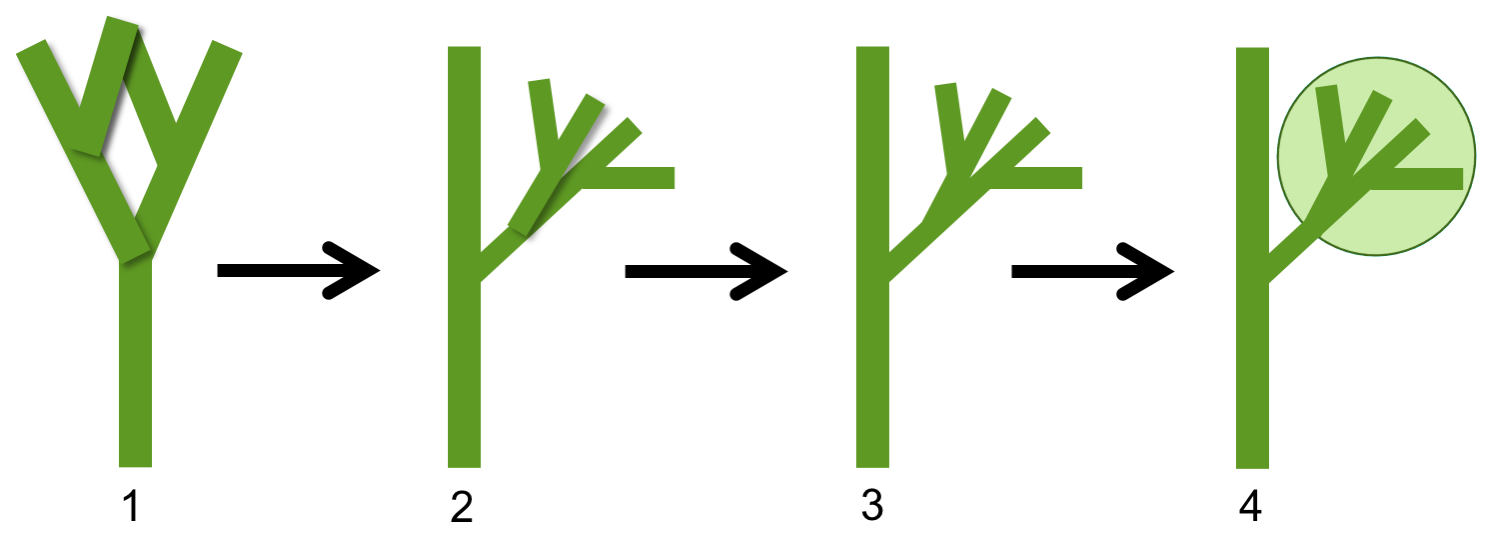
Telome theory for origin of megaphylls (euphylls). The steps in the telome theory, which is a theory for the origin of euphyllophyte leaves, is as follows: 1. Axis with equal dichotomous, three-dimensional branching. 2. Axis with unequal branching; one branch is dominant whereas the others form subordinate branching systems. 3. Lateral branching systems become flattened (planar). 4. Webbing develops between branches, forming a leaf. Credit: Diagram by E.J. Hermsen (DEAL). After fig. 1 in Rothwell et al. (2014), among other sources.
Microphylls vs. Megaphylls
Microphylls | Megaphylls | |
---|---|---|
Alternate name | Lycophylls | Euphylls |
Plant group | Lycophytes | Euphyllophytes |
Evolution | From enations | From branching systems |
Number of independent originations | One | More than one |
Leaf stalk | Absent, leaf scale-like | Absent or present |
Leaf venation | Usually one unbranched vein | Variable, venation often branching |
Associated stele type | Protostele | Usually siphonostele or eustele |
Associated leaf gaps | Absent | Usually present |
Selected references & further reading
Note: Free access is provided by the publisher for items marked with a green asterisk. Note that restrictions may apply for reuse.
Academic articles & book chapters
* Doyle, J.A. 2013. Phylogenetic analyses and morphological innovation in land plants. In B.A. Ambrose and M. Purugganan (eds.), The Evolution of Plant Form. Annual Plant Reviews 45: 1–50. https://doi.org/10.1002/9781119312994.apr0486
* Melo, E., C.A. Cid Ferreira, and R. Gribel. 2019. A new species of Coccoloba P. Browne (Polygonaceae) from the Brazilian Amazon with exceptionally large leaves. Acta Amazonica 49. https://doi.org/10.1590/1809-4392201804771
* Rothwell, G.W., S.E. Wyatt, and A.M.F. Tomescu. 2014. Plant evolution at the interface of paleontology and developmental biology: An organism-centered paradigm. American Journal of Botany 101: 899–913. https://doi.org/10.3732/ajb.1300451
Schneider, H., A.R. Smith, and K.M. Pryer. 2009. Is morphology really at odds with molecules in estimating fern phylogeny? Systematic Botany 34: 455–475. https://doi.org/10.1600/036364409789271209
Tomescu, A.M.F. 2008. Megaphylls, microphylls and the evolution of leaf development. Trends in Plant Science 14: 5–12. https://doi.org/10.1016/j.tplants.2008.10.008
Tryon, R. 1960. A glossary of some terms relating to the fern leaf. Taxon 9: 104-109. https://doi.org/10.2307/1216244
* Vasco, A., R.C. Moran, and B.A. Ambrose. 2013. The evolution, morphology, and development of fern leaves. Frontiers in Plant Science 4, Article 345. https://doi.org/10.3389/fpls.2013.00345
Books, textbooks & manuals
Coulter, J.M., C.R. Barnes, and H.C. Cowles. 1910. A Textbook of Botany for Colleges and Universities, vol. I. Morphology and Physiology. American Book Company, New York. Read free online at Internet Archive: https://archive.org/details/textbookofbotan01coul
Ellis, B., D. C. Daly, L. J. Hickey, K. R. Johnson, J. D. Mitchell, P. Wilf, and S. L. Wing. 2009. Manual of leaf architecture. Comstock Publishing Associates, Cornell University Press, Ithaca, New York.
Esau, K. 1977. Anatomy of Seed Plants, 2nd ed. John Wiley & Sons, Inc., New York.
Evert R.F., and S.E. Eichhorn. 2013. Raven Biology of Plants, 8th ed. W.H. Freeman and Co., New York, New York.
Foster, A.S., and E.M. Gifford. 1974. Comparative Morphology of Vascular Plants, 2nd ed. W.H. Freeman and Co., San Francisco.
Harris, J.G., and M.W. Harris. 2001. Plant Identification Terminology, an Illustrated Glossary, 2nd ed. Spring Lake Publishing, Spring Lake, Utah.
Leaf Architecture Working Group (A. Ash, B. Ellis, L.J. Hickey, K. Johnson, P. Wilf, and S. Wing). 1999. Manual of Leaf Architecture: Morphological description and categorization of dicotyledonous and net-veined monocotyledonous angiosperms. Smithsonian Institution, Washington, DC.
Simpson, M.G. 2010. Plant Systematics, 2nd ed. Academic Press, Burlington, Massachusetts.
Willis, K.J., and J.C. McElwain. 2002. The Evolution of Plants, 2nd ed. Oxford University Press, U.K.
Websites
* Cofrin Center for Biodiversity Herbarium, University of Wisconsin-Green Bay. Pteridophytes of Wisconsin: Ferns and Fern Allies: Glossary of selected pteridophyte terminology. https://www.uwgb.edu/biodiversity/herbarium/pteridophytes/pteridophyte_glossary01.htm
* Plant Anatomy Laboratory: Micrographs of plant cells and tissues, with explanatory text (J.D. Mauseth): http://www.sbs.utexas.edu/mauseth/weblab/
* USDA Forest Service: Fern Structure. https://www.fs.fed.us/wildflowers/beauty/ferns/structure.shtml
Content usage
Usage of text and images created for DEAL: Text on this page was written by Elizabeth J. Hermsen. Original written content created by E.J. Hermsen for the Digital Encyclopedia of Ancient Life that appears on this page is licensed under a Creative Commons Attribution-NonCommercial-ShareAlike 4.0 International License. Original images and diagrams created by E.J. Hermsen are also licensed under Creative Commons Attribution-NonCommercial-ShareAlike 4.0 International License.
Content sourced from other websites: Attribution, source webpage, and licensing information or terms of use are indicated for images sourced from other websites in the figure caption below the relevant image. See original sources for further details. Attribution and source webpage are indicated for embedded videos. See original sources for terms of use. Reproduction of an image or video on this page does not imply endorsement by the author, creator, source website, publisher, and/or copyright holder.
Adapted images. Images that have been adapted or remixed for DEAL (e.g., labelled images, multipanel figures) are governed by the terms of the original image license(s) covering attribution, general reuse, and commercial reuse. DEAL places no further restrictions above or beyond those of the original creator(s) and/or copyright holder(s) on adapted images, although we ask that you credit DEAL if reusing an adapted image from the DEAL website. Please note that some DEAL figures may only be reused with permission of the creator(s) or copyright holder(s) of the original images. Consult the individual image credits for further details.
First released 2 June 2020; last updated 26 January 2021.