Chapter by:
Jansen A. Smith, Paleontological Research Institution, Ithaca, New York
This chapter was first publicly shared on April 15, 2020. Chapter title updated on August 14, 2024.
Chapter citation:
J.A. Smith. 2020. Introduction to Conservation Paleobiology. In: The Digital Encyclopedia of Ancient Life. https://www.digitalatlasofancientlife.org/learn/conservation-paleobiology/
Chapter contents:
Conservation Paleobiology ←
– 1. Foundations in Conservation Biology
– 2. Approaches in Conservation Paleobiology
–– 2.1 Near-time Approaches
–– 2.2 Deep-time Approaches
– 3. Deliverables from Conservation Paleobiology
– 4. Barriers to Applying Conservation Paleobiology
Above image: An accumulation of sea shells, in a formation called a chenier, in the northern Gulf of California. These shells pre-date dam construction and water diversion along the Colorado River and provide a means to evaluate the community prior to widespread ecosystem change. Photo by Jansen Smith.
Introduction
Conservation paleobiology is a new and growing interdisciplinary field that applies the data and methods of paleontology to the conservation and restoration of present-day biodiversity and ecosystem services (the benefits people derive from the world around us). This definition of the field carries two important implications. First, by being interdisciplinary, conservation paleobiology incorporates data, theories, concepts, and practitioners from different disciplinary backgrounds. These include elements of paleontology, sedimentology, community ecology, restoration ecology, and more. Likewise, conservation paleobiology can be considered as one sub-field under the broader conceptual umbrella of conservation science.
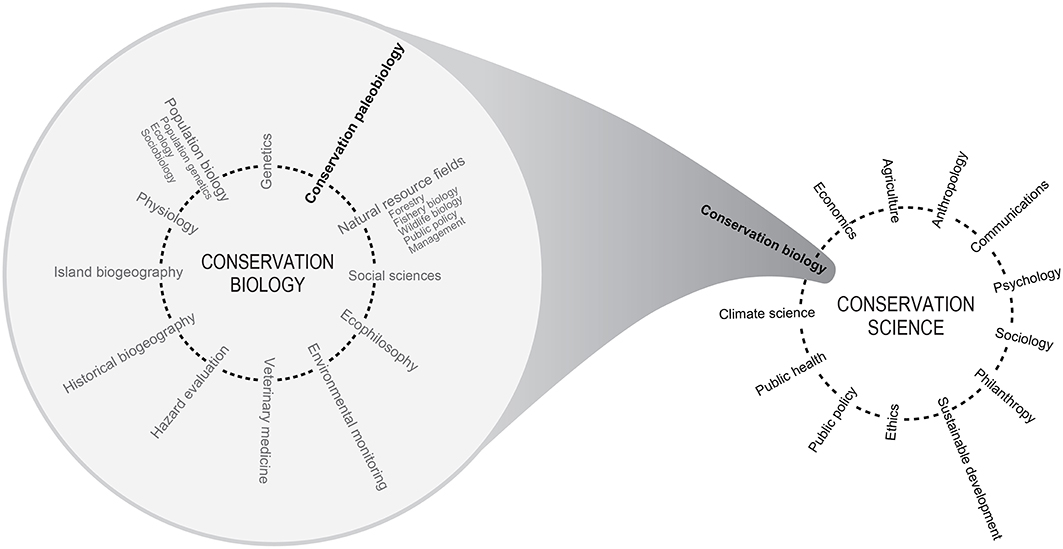
"The expanding domain of conservation science. The left diagram illustrates the contribution of conservation paleobiology to Soule's (1985) synthetic, cross-disciplinary conservation biology. The diagram on the right depicts how Soule's conservation biology fits into Kareiva and Marvier's (2012) broader view of conservation science. Adapted from Peter Kareiva and Michelle Marvier, What Is Conservation Science? BioScience (2012) 62(11):962–969, by permission of the American Institute of Biological Sciences." Image from Dietl (2016) in Frontiers in Ecology and Evolution (open access); Creative Commons Attribution 4.0 International license.
Whether at the finer scale of conservation paleobiology or under the umbrella of conservation science, the integration of elements from numerous fields and sub-fields is in recognition of the fact that ecosystems are complex systems, and, in conservation and restoration, many stakeholders and their goals must be considered.
This brings us to the second important implication of the definition: conservation paleobiology is a goal-oriented, value-driven field. Broadly, the goals are to conserve and restore present-day biodiversity and ecosystem services. By extension of these goals, value is placed on biodiversity and ecosystem services: they are considered to be “good.” As we will explore in the section on the broader field of conservation biology, these goals are often much more specific and are often motivated by the stakeholders involved in the decision-making process. Nonetheless, research in conservation science strongly adheres to the scientific process. Research questions and experimental designs do cater to specific areas of need or interest, but when it comes to collecting, analyzing, and interpreting the data, bias plays no part in the process. Conservation is a science-driven pursuit and applies the best available information to the decision-making process.
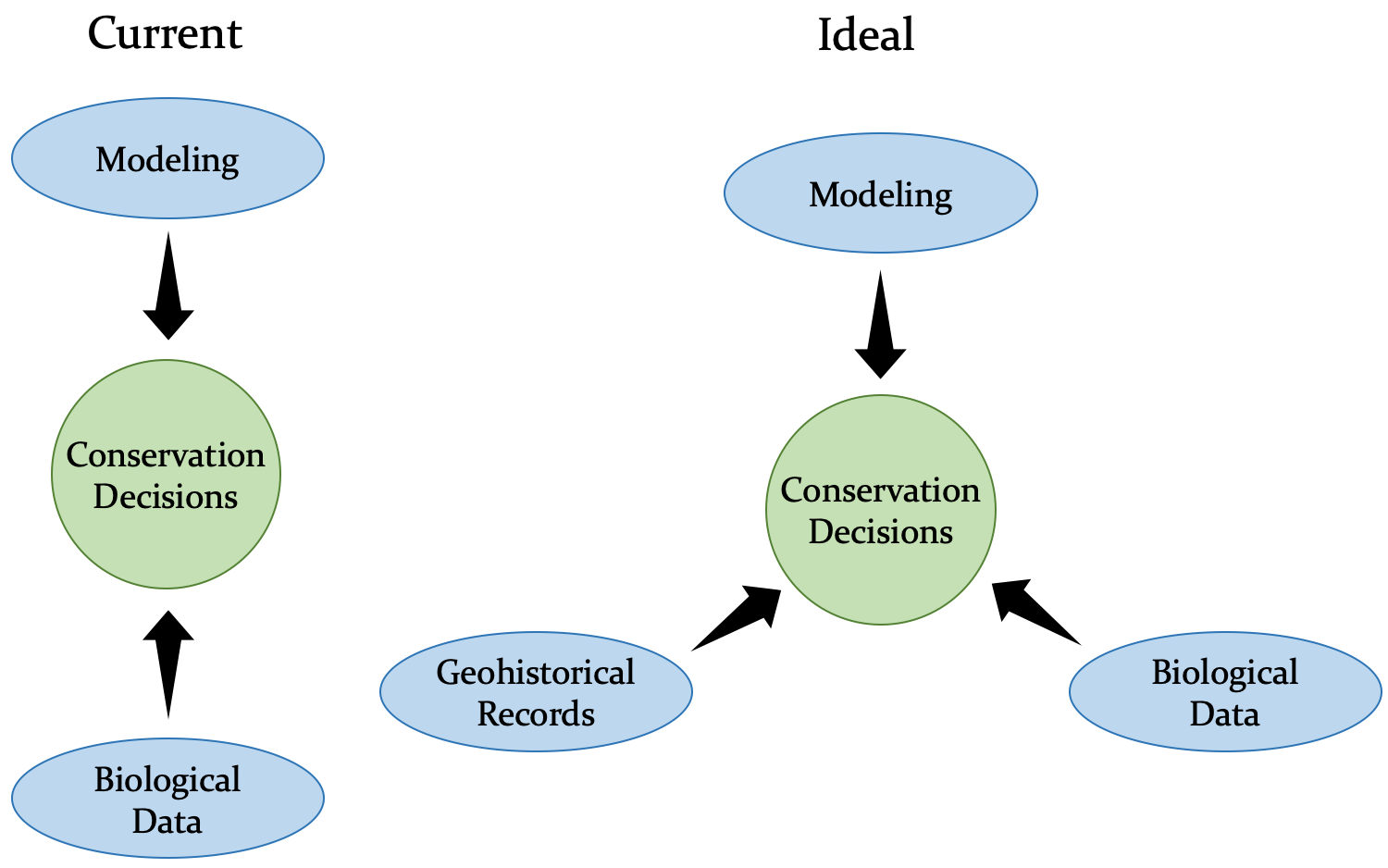
Currently (left), conservation decisions are made using data from computer models and observations or experiments with living organisms. Ideally (right), geohistorical records would also be used to supplement the decision making process by adding a longer temporal perspective.
Directly or indirectly, studies in conservation paleobiology use the past history of life to contextualize the world around us today and this context can be applied to improve the decisions made in conservation and restoration practice. Using the past history of life can be challenging because it requires that we think about long-acting processes and timescales well beyond the duration of a single human life. It requires geohistorical thinking.
To think geohistorically, it is important to first recognize that today represents only a very brief moment in time. Compared to the amount of time that has already passed, and will come to pass in the future, the current moment represents less time than the blink of an eye as compared to the duration of your life. As you begin to appreciate the vastness of time, you can also start to understand more thoroughly that the world is constantly changing. By contrast, compared to many human processes and concerns, the natural world can seem constant. On human timescales, ecosystems can appear stable: a forest from your childhood is still a forest today. This appearance of stability has driven many conservation and restoration goals, as people have sought to maintain, or regain, a particular set of ecosystem conditions based on their idea of what is “natural.” Failure to recognize change in the world will prime these efforts to fail, as the world continues to change—with or without human driven climate change. Embracing a geohistorical pattern of thought means incorporating dynamism into our restoration and conservation efforts. It means recognizing that the past provides context for the world around us today, just as the world around us today will be our frame of reference for the future. Geohistorical thinking, because it considers events and processes beyond the scope of human observation, requires data sources that are outside the standard purview of conservation science. These data sources often take the form of geohistorical records.
Geohistorical Records
Geohistorical records provide environmental or biological information about the past and include sediment cores, ice cores, tree ring series, fossils, and other natural archives. These records are unique in that they can contain information from vast periods of time (e.g., centuries, millennia, millions of years, and longer) and provide insight into how long-acting processes unfold. For example, ice cores provide highly detailed records of atmospheric gas concentrations over the last 800,000 years. Using these concentrations as proxies, we can reconstruct climate (e.g., temperature) cycles for this duration of time. It is by comparison to these records that we know today’s climate is deviating from the cyclicity experienced on Earth during the preceding 800,000 years.
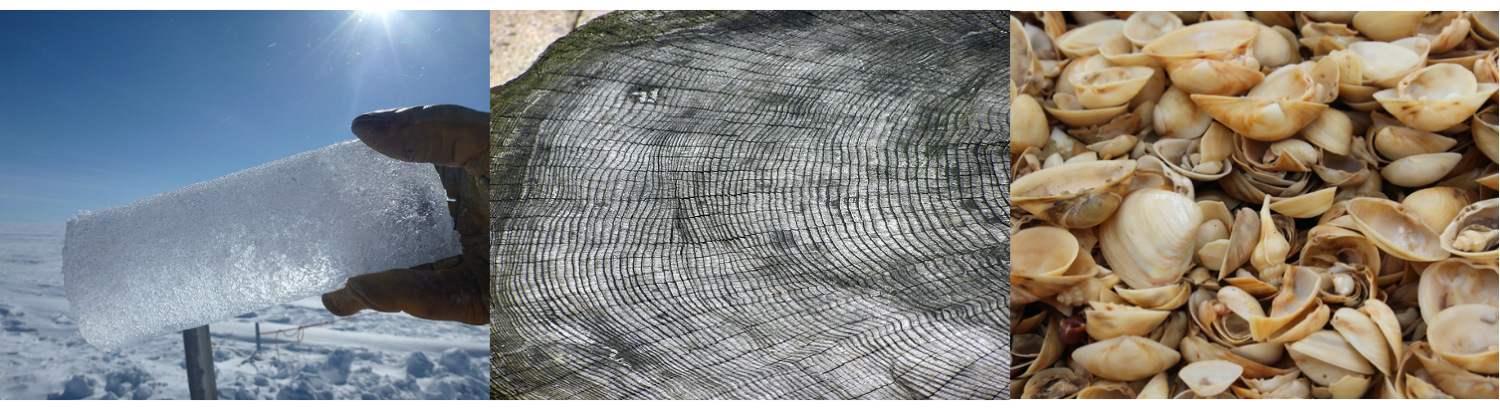
A few of the different types of geohistorical records: an ice core (left), tree rings (center), and fossils (right). Left image by NASA's Goddard Space Flight Center/Ludovic Bucker (public domain). Center image by Arpingstone (public domain). Right image by Jansen Smith.
By using proxies from ice cores and other geohistorical records to understand how the environment has changed over time (learn more in the section on Paleoenvironmental Reconstruction), we can use evidence from fossils to begin to evaluate how life has responded to different scenarios in the past. With this approach, which is commonly used in deep-time conservation paleobiology, the fossil record can be considered a natural laboratory, where thousands and thousands of experiments have been running throughout the history of life. Alternatively, in near-time conservation paleobiology, species found in the fossil record are often still living and, because this approach focuses on the relatively young fossil record, can be used in more direct comparisons to the modern world. Simply put, the near-time approach provides spatial and temporal context for the distributions and interactions of species in modern ecosystems and the deep-time approach helps us build potential scenarios for how species and ecosystems are changing in response to changing environmental conditions, some of which may impact modern times or have the potential to arise in our future.
Informative as they may be, it can be challenging to interpret geohistorical records because they tend to be time-averaged and taphonomically altered (learn more about the concepts time-averaging and taphonomy). Time-averaging occurs due to the mixing of fossils from organisms that did not live at the same time, and may have lived a year, a decade, a century, or a millennium apart. For a more familiar example, we can consider a cemetery. If you were to walk through a cemetery, you would notice that the dates on the tombstones are from the 2000s, 1900s, 1800s, and maybe even further back in time. Though some of these people lived in overlapping times, a person who lived in the 2000s and another from the 1800s almost certainly did not live at the same time. This fundamental attribute of geohistorical records must be considered when data from these records are interpreted and applied.
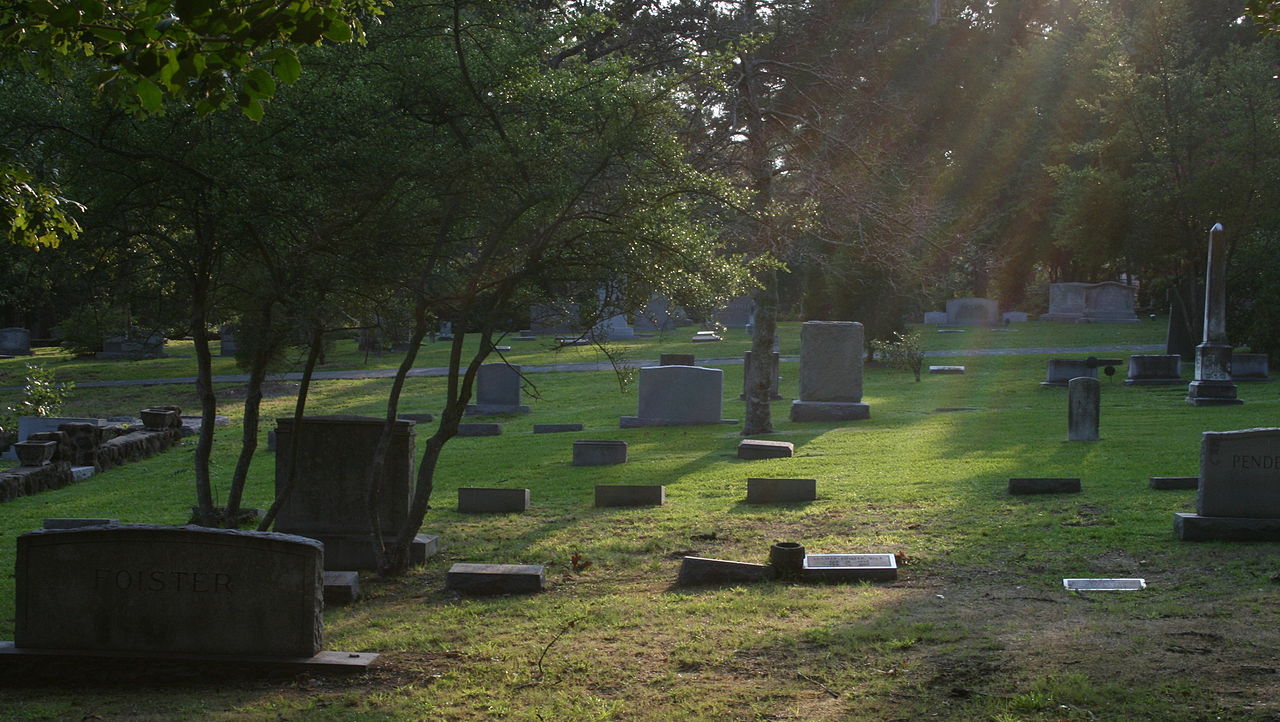
Imagine walking through the cemetery and noting the different times when the people buried there were alive; conceptually you would be noting the degree to which the cemetery is time-averaged. Image by Ildar Sagdejev; GNU Free Documentation License.
Much like time averaging, taphonomic alteration also needs to be considered when interpreting and applying data from geohistorical records. Taphonomy refers to the suite of processes that can alter the remains of organisms from their time of death to their time of study. These processes can include consumption or scavenging of the remains, breakage by environmental factors, removal from the record by erosional processes, or distortion by geological processes, to name a few. As these processes can remove important data, it is important to understand whether, and to what extent, the geohistorical record has been altered before making conservation or restoration decisions based on these records. Though taphonomy (and time-averaging) can make it more challenging to interpret the geohistorical record, it does not preclude it from being useful in conservation and restoration practice.
Applying Geohistorical Records in the Galapagos
The Galápagos Islands (Ecuador) have become famous for the unique life found on the numerous small volcanic islands in this archipelago. Many of the life forms on these islands are not found on any other place on Earth—making them endemic to the Galápagos—and have been studied extensively to understand evolutionary and ecological processes. For example, the islands are home to “Darwin’s finches,” a group of finch species of diverse sizes and ecologies that evolved from a single ancestral species (learn more about Darwin's finches). As with many other island systems, the Galápagos ecosystems are carefully monitored to conserve and restore their uniqueness. As such, everything from the number of people visiting the islands to the plants and animals living on them is closely watched.
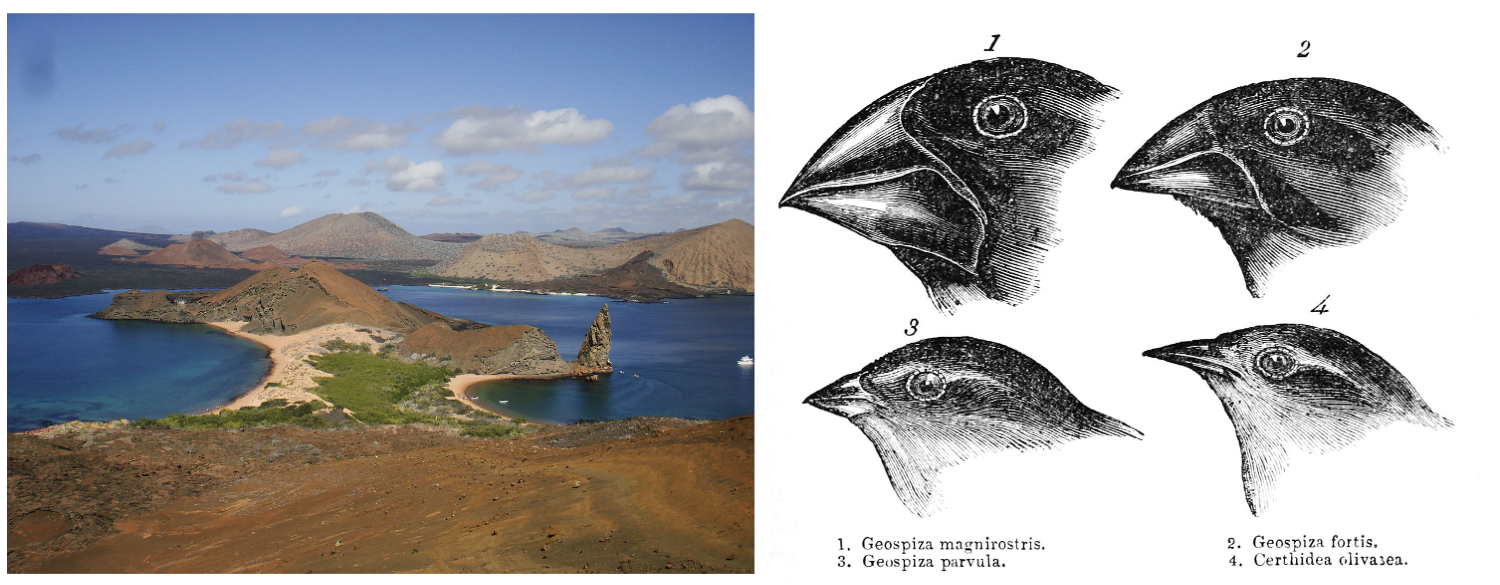
Bartolomé Island showing the volcanic landscape common on the Galapagos Islands and a handful of the finch species found on the islands. Left image by Pete; Creative Commons Attribution-Share Alike 2.0 Generic license. Right image by John Gould (public domain).
Among these efforts have been attempts to remove non-native species so as to ensure endemic species can thrive. These efforts tend to be based on observations and scientific studies of life on the islands. In the Galápagos, the first human presence on the islands is believed to have been in 1535 and this date is used as a frame of reference for conservation and restoration decisions. If a species was present prior to 1535, it is considered native. If the species was brought to the islands by people (either intentionally or unintentionally), it is considered non-native and will be removed from the islands (at least in theory). Although this decision-making process is quite clear, it can be a challenge to determine when a species arrived on the islands because few studies and observations date back far enough to make this determination. Instead, as discussed by Jacqueline van Leeuwen and her colleagues (2008), the information used to make decisions can be from incomplete or unreliable sources. For example, for poorly studied species, data on their closest relatives is often used as a proxy, in place of actual data on the species of interest. In some cases, species-specific data does exist but only in museum collections (i.e., herbariums) from sporadic times and places. Another common source for determining native status is the perception of a species by people; if a species is considered "weedy" it is usually also considered non-native. Thus, the classification of a species as native or non-native is based on the best available evidence, but the best available evidence is not always complete.
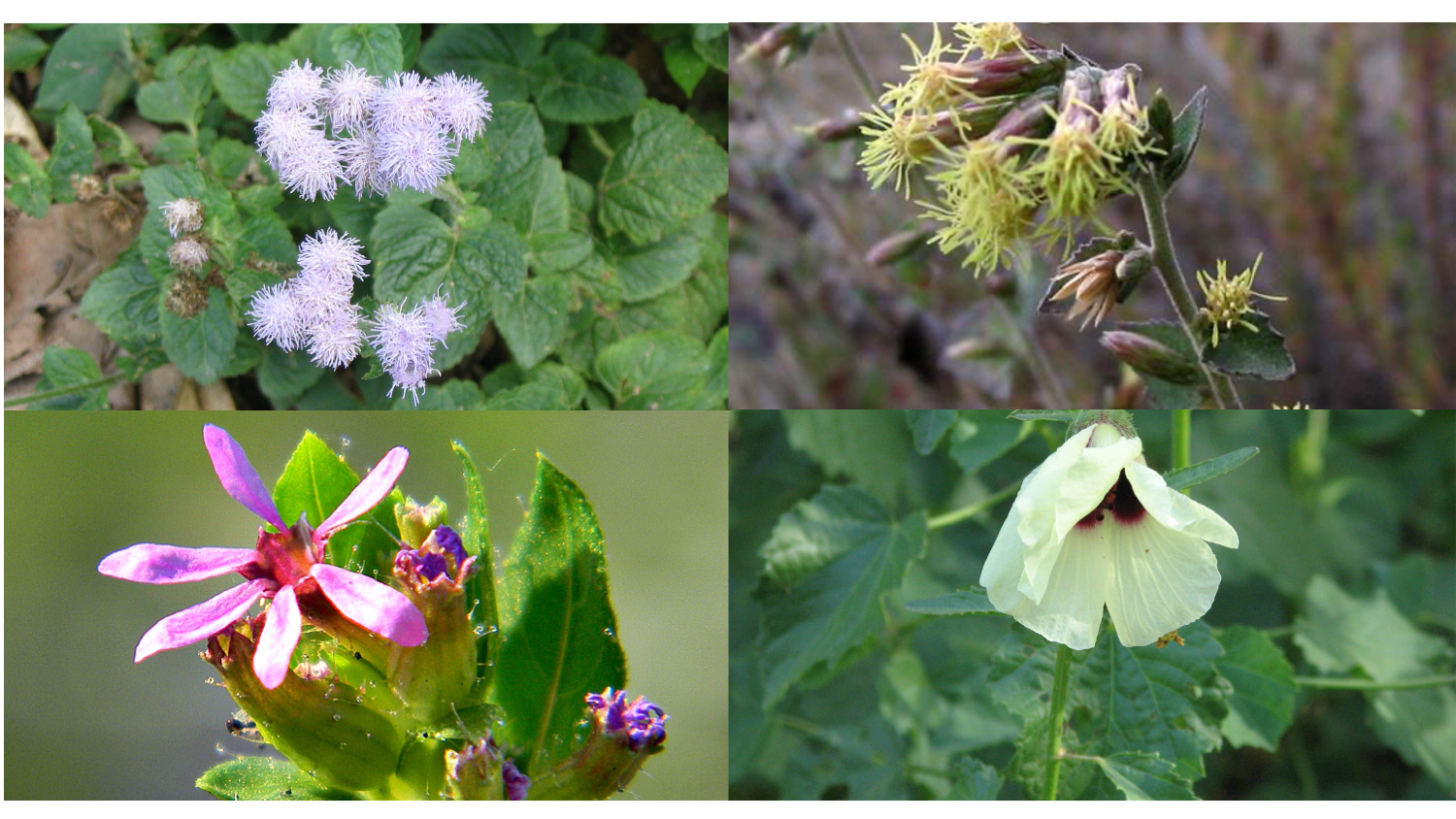
Four of the plants found to be native to the Galapagos based on pollen records predating human arrival on the islands. Top left: Ageratum conyzoides by Minghong (Creative Commons Attribution-Share Alike 3.0 Unported license); Top right: Brickellia (public domain); Bottom left: Cuphea carthagenensis by Bob Peterson (Creative Commons Attribution-Share Alike 2.0 Generic license); Bottom right: Hibiscus diversifolius by Melburnian (GNU Free Documentation license).
Geohistorical records can help fill in the gaps for some species, particularly plants in the Galápagos. On several of the islands, lowland areas are home to bog ecosystems and have been for thousands of years. In these bogs, pollen from plants on the islands gets trapped and preserved in the sediments and, because the shape and size of pollen is often plant-specific, it can be used to evaluate the presence of specific plants. By examining cores taken from the sediments of these bogs, the team of researchers led by van Leeuwen were able to improve the classification of several plants on the Galápagos Islands. Six plants (Ageratum conyzoides, Borreria laevis/Diodia radula–type, Brickellia diffusa, Cuphea carthagenensis, Hibiscus diversifolius, and Ranunculus flagelliformis) that had been thought to be non-native, or were considered to be doubtfully native, were abundantly represented by pollen in the sediment cores. Importantly, radiocarbon dating shows that the pollen was present in the cores for thousands of years, well beyond early human contact in 1535.
In this example from the Galápagos, geohistorical pollen records likely changed the restoration actions that would have been undertaken. Without the pollen data, the six plants identified as native by van Leeuwen and her colleagues would still have been considered non-native. Those plants would likely have been removed from the islands, even though they were native! Geohistorical records can have important implications for conversation and restoration decision making.
Concept check: See what you know!
What implications are carried by the definition of "conservation paleobiology?"
There are two important implications: (1) conservation paleobiology is interdisciplinary and draws data, theories, and concepts from related fields; and, (2) conservation paleobiology is a goal-oriented, value-driven field.
What are ecosystem services?
Ecosystem services are the benefits that people gain from ecosystems. These include supplying food through wild game; purifying our drinking water; sequestering carbon from the atmosphere; and many more important benefits.
Name three types of geohistorical data.
Geohistorical records include: sediment cores, ice cores, tree ring series, fossils (including pollen), and other natural archives.
What role did geohistorical records play in the Galápagos?
As discussed in this chapter, the pollen record clearly indicated that six plant species previously thought to be non-native in the Galápagos were in fact native.
What two attributes of geohistorical data must be considered before making any decisions?
These attributes are time-averaging (the mixing of organismal remains from individuals not living at the same time) and taphonomy (the processes that destroy and distort geohistorical records).
References and Further Reading
Bjornerud, M. 2018. Timefulness: how thinking like a geologist can help save the world. Princeton University Press, Princeton, New Jersey, 219 pp.
Dailey, G. 2004. Geohistorical inquiry: Connecting place and time and critical thinking. USA. ESRI. Education Program 13 p.
Dietl, G. P., S. M. Kidwell, M. Brenner, D. A. Burney, K. W. Flessa, S. T. Jackson, and P. L. Koch. 2015. Conservation paleobiology: leveraging knowledge of the past to inform conservation and restoration. Annual Review of Earth and Planetary Sciences, 43: 79-103.
Kravitz, G. 2014. The geohistorical time arrow: from Steno's stratigraphic principles to Boltzmann's past hypothesis. Journal of Geoscience Education, 62: 691-700.
Tyler, C. L., and C. L. Schneider. 2018. Marine Conservation Paleobiology. Springer, Cham, Switzerland, 261 pp.
van Leeuwen, J. F., C. A. Froyd, W. O. van der Knaap, E. E. Coffey, A. Tye, and K. J. Willis. 2008. Fossil pollen as a guide to conservation in the Galápagos. Science, 322: 1206-1206.
Usage
Unless otherwise indicated, the written and visual content on this page is licensed under a Creative Commons Attribution-NonCommercial-Share Alike 4.0 International License. This page was written by Jansen A. Smith. See captions of individual images for attributions. See original source material for licenses associated with video and/or 3D model content.