Chapter contents:
Evolution and the Fossil Record
– 1. Natural selection
– 2. Species and species concepts
– 3. Speciation
– 4. Punctuated equilibria and stasis
–– 4.1 Videos about punctuated equilibrium and stasis
– 5. Macroevolution ←
–– 5.1 Hierarchies
–– 5.2 Species selection
–– 5.3 Abiotic vs. biotic causes of macroevolution
–– 5.4 Evolutionary radiations
What is macroevolution?
The meaning of the term “macroevolution” has shifted over time. Indeed, early definitions do to not necessarily make much sense in light of our current understanding of evolution, yet are still worth considering to show how the field itself has evolved. Here we will consider usage of the term macroevolution in a few key works, as well as present a definition of macroevolution that we endorse.
The term “macroevolution” seems to have been coined by a Russian entomologist named Yuri Filipchenko (1927) in “Variabilität und Variation.”
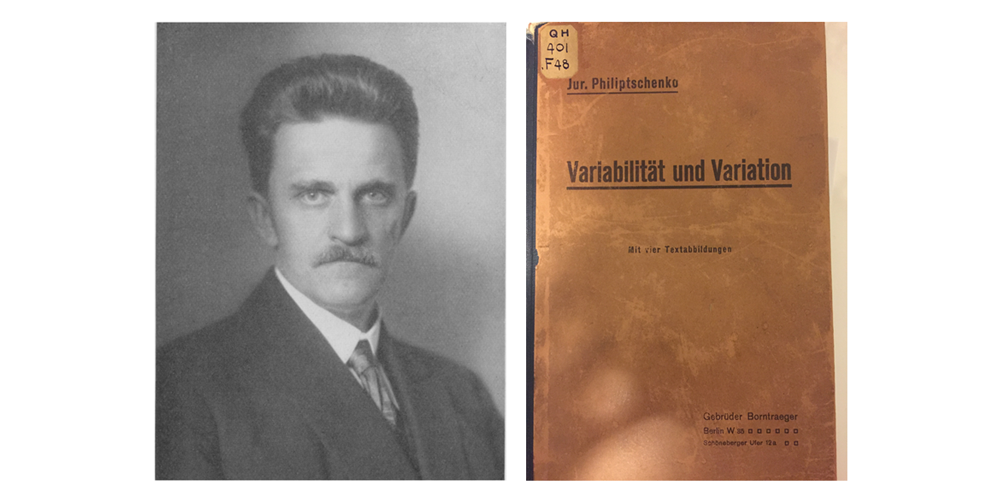
Left: Photograph of Filipchenko (Wikimedia Commons; public domain). Right: front cover of Variabilität und Variation; image by "Philibenl" (Wikimedia Commons; Creative Commons Attribution-Share Alike 4.0 International license).
In this work, Filipchenko used “macroevolution” to refer to the origins of characters that define genera and other groups above the species level. This perspective posited that there is something about the origins of characters that define Linnean taxa (genera, families, or orders, etc.) that is different from the origins of characters that define species.
Filipchenko believed in a macroevolutionary process that could create novel features in genera and other higher taxa. This view of macroevolution has aspects in common with the views of Richard Goldschmidt, most famously developed in his 1940 book, “The Material Basis of Evolution.” Goldschmidt argued that the origins of new species involved macromutations and these were qualitatively different from the types of genetic changes that occurred within species. He focused on the discontinuity he saw between intra-specific and inter-specific change. In contrast, Filipchenko focused on the origins of characters rather than the group possessing them. It is worth considering that both Filipchenko's and Goldschmidt's concepts of macroevolution are based on processes (e.g., macromutations) rather than the patterns that result from those processes.
Evolutionary patterns represent identified similarities or differences in features among biological entities. These are recognized by analysis and description of the features, as well as how those features change in the entities over time or across geographic space. Evolutionary processes are the forces or mechanisms that produce the evolutionary patterns. As an example, differences over time in the rate of survival of the offspring of one lineage versus another is a pattern. The biological process of natural selection might explain such an observed pattern.
The definition of macroevolution given in Filipchenko's “Variabilität und Variation” is not consistent with our modern understanding of how evolution works. Evolution does not cause one genus to give rise to another through a process of "generification." Genera and other higher taxa (e.g., families, orders, classes, and phyla) are not real in any biological sense. Instead, higher taxa are simply human constructs that are useful for communicating biological information, especially relative degrees of evolutionary relationship (see Hendricks et al., 2014). Considerations of the meaning of higher taxa in a phylogenetic framework have been discussed at length elsewhere and the reader is encouraged to explore the foundational books by Willi Hennig (1966), Niles Eldredge and Joel Cracraft (1980), and Ed Wiley (1981).
Higher taxa reflect differential patterns of species-level evolution and extinction that have occurred over millions of years. This is why members of closely-related genera look different from each other, but nevertheless share many features in common. For example, humans (genus Homo) and chimpanzees (genus Pan) share numerous physical and genetic similarities, but are also immediately recognizable as different. These differences become less distinct, however, when we consider ancient, extinct stem-group relatives of humans, some of which were much more chimp-like in appearance.
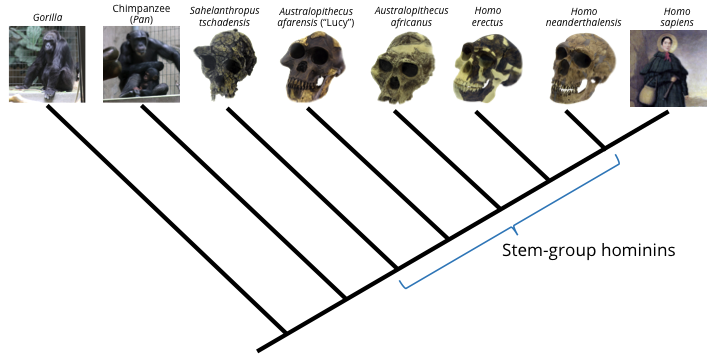
Among living species, humans (Homo sapiens) are most closely related to chimpanzees (Pan troglodytes). There are many extinct hominid species (stem-group hominids), however, that are more closely related to modern humans than they are to chimps. Some of these probably had appearances and behaviors that were very chimp-like, blurring the distinctions that are apparent today between living chimps and humans.
A popular pattern-based definition for macroevolution is "all evolution above the species level." For a variety of reasons, however, it is problematic to connect this type of definition to evolutionary processes (see above). The same is also true of morphological characteristics, which don't exist independently of the organisms that posses them (rendering Filipchenko's views problematic). Organisms must be thought about as integrated wholes, rather than just a collection of characteristics that are parsed out and described by biologists and paleontologists.
Similarly, species might be thought about as integrated wholes (they are comprised of individual organisms that are nearly genetically identical) until they are not. Cladogenesis involves the splitting of lineages as a consequence of speciation (covered earlier in this chapter). Speciation and lineage splitting is what macroevolution is really all about. Niles Eldredge (1979) characterized this as a "taxic" view of evolution (which we endorse) and contrasted it with the older "transformational" view of evolution, which focused on the gradual change of one species into another over long periods of geological time. As discussed in the section on punctuated equilibria, the fossil record provides very little support for such a transformational model of evolution.
The Modern Synthesis & Tempo and Mode of Evolution
Another key figure in evolutionary biology who often invoked the term “macroevolution” was paleontologist George Gaylord Simpson. Especially relevant is his classic 1944 book “Tempo and Mode in Evolution” which—along with Theodosius Dobzhansky’s (1937) “Genetics and the Origin of Species” and Ernst Mayr’s (1942) “Systematics and the Origin of Species”—is considered a foundational text of the so-called Modern Synthesis (coined by Julian Huxley in 1942) of the middle of the 20th century.
The Modern Synthesis wove together several major threads of biological thought into a more cohesive whole. In particular, Darwin's views about natural selection became integrated with increasing awareness about genetic inheritance and the importance of mutations in creating the novelty upon which selection acts. Dobzhansky contributed the perspective of genetics, Mayr presented views on the nature of species and how they originate, and Simpson offered a deep-time perspective about evolution given the evidence from the fossil record. For a detailed discussion of these three books, their significance to evolutionary biology, and the Modern Synthesis, see Eldredge’s (1985) book “Unfinished Synthesis.”
We will focus here on the evolutionary perspective of Simpson (1944), who held that macroevolution involved “the rise and divergence of discontinuous groups” and occurred at the levels of species and genera. One of Simpson's most important contributions was that he was the first scientist to convincingly document that rates of evolution varied through time and were not constant. A focus on evolutionary rate is of course critical to macroevolution, and the primary way that Simpson expressed this was his concept that there were different tempos of evolution. He recognized three distinct types: tachytelic, horotelic, and bradytelic.
Tachytelic groups are those with high evolutionary tempos. Simpson argued that there were several times in the history of life when tachytelic evolutionary tempos prevailed, for instance, within mammals in the early part of the Cenozoic when they underwent major radiation.
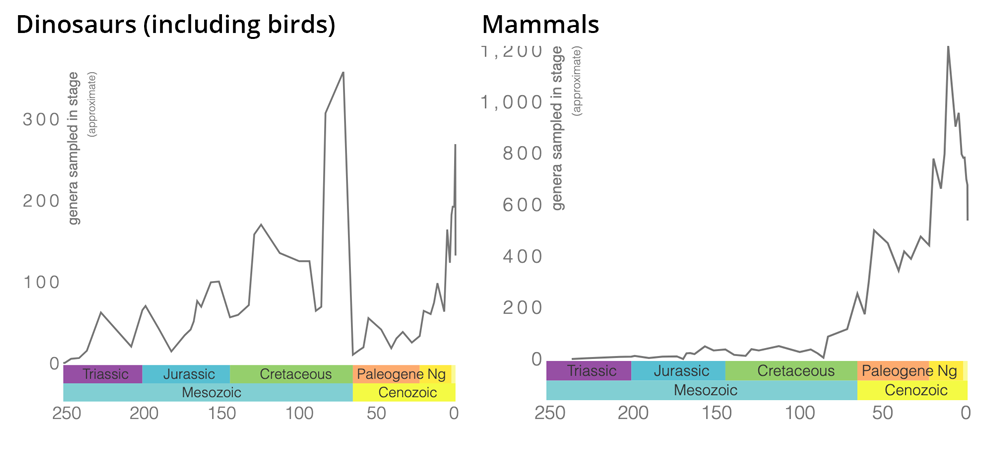
Patterns of dinosaur and mammal genus-level diversity from the Mesozoic to today. Note the explosive diversification of mammals following the extinction of non-avian dinosaurs at the end of the Cretaceous period 66 million years ago. Graphs were produced using the Paleobiology Database Navigator. Note that the Y-axes are different in each graph.
Further, studying such rapidly diversifying groups provided important insights into evolution. However, because evolution was happening so rapidly in such groups, Simpson argued that figuring out the processes involved and sequence of events occurring would be difficult given the level of completeness typically preserved in the fossil record. Instead, Simpson made the clever connection that while it would be hard to study evolution when tachytelic tempos prevailed, it would, by contrast, be much easier to study slowly evolving lineages that displayed bradytelic tempos of evolution. Simpson further argued that if paleontologists could determine what was causing slow evolution in bradytelic groups, then they would be able to recognize how opposite phenomena could produce tachytelic evolutionary tempos.
Although the terms bradytely and tachytely are not used much today, bradytelic lineages are still the focus of important evolutionary studies for precisely the reasons Simpson identified. Such lineages, when extant, are often referred to as “Living Fossils.” They include groups that have undergone seemingly little morphological change over millions of years. Examples include ginkgo trees, coelacanths, and horseshoe crabs. Compare, for example, the specimens below of modern and fossil horseshoe crabs that are separated in time by over 150 million years.
A modern specimen of the horseshoe crab Tachypleus tridentatus from Hong Kong. Specimen is from the research collections of the Paleontological Research Institution. Approximate length (not directly measured) is 10 cm.
Fossil specimen of the horseshoe crab Mesolimulus walchi from the Jurassic Solnhofen Limestone of Germany (PRI 50591). Specimen is on display at the Museum of the Earth, Ithaca New York. Length of specimen is approximately 14 cm.
A Digital Atlas Virtual Exhibit called "Survivors: Up Close with Living Fossils" may be accessed here. It is based on a physical exhibit that was developed by Paleontological Research Institution staff and displayed at the Museum of the Earth in 2019.
A Modern Definition of Macroevolution
Lieberman and Eldredge (2014) defined macroevolution as “the patterns and processes pertaining to the birth, death, and persistence of species” and we adopt this definition here. Thus, phenomena such as punctuated equilibria and the mechanisms that lead to species stasis are directly within the purview of macroevolution. Researchers who study macroevolution investigate phenomena that cause speciation and extinction and the patterns that result in the fossil record. They might also study the factors that cause differences in degrees of morphological change during diversification events. These are just a couple of examples, and many other types of biological and paleontological research could also be referred to as macroevolutionary in scope.
One additional point of discussion: the aforementioned definition from Lieberman and Eldredge (2014) does not include taxa above the species-level (genera, families, etc.) because there are not evolutionary processes that operate above the species-level. It is true that a comparative study of the long-term patterns of individual species in some species-rich clade (e.g., Trilobita) could provide insights into macroevolution, but that is only because such a clade contains a large number of species that share a common evolutionary ancestry. As a result, the evolutionary dynamics within such a clade represent the sum of attributes across all the individual species within the clade, including the events (e.g., time of origin and extinction) that shaped their individual histories.
Does Microevolution = Macroevolution?
One of the big debates in evolutionary biology centers around whether there is a substantive difference between microevolution, defined as changes within species, and macroevolution, defined as changes among species. At its core, this debate centers around whether macroevolutionary change simply represents the summation of countless microevolutionary changes, or if it involves fundamentally disconnected and different processes beyond natural selection, genetic drift, etc. The architects of the modern synthesis weighed in on this topic in the mid-20th century, and their viewpoints are perhaps best expressed by Dobzhansky (1937), who posited that scientists might as well assume that macroevolution reflected the byproduct of extrapolated microevolution. Simpson (1944) did agree with this perspective, but saw a qualitative difference between “megaevolution” and “micro”- and “macroevolution”; he later abandoned this view (Simpson, 1953), however, and held that all evolution was extrapolated “microevolution.” Others such as Stephen Jay Gould have disagreed and argued that micro- and macroevolution are disconnected.
The perspective we present here accepts neither the standard modern synthesis view nor Gould’s view. Instead, our view aligns with the outlook presented by Niles Eldredge and Elisabeth Vrba. They argued that the microevolution vs. macroevolution debate actually misses the point and is no longer productive. This is because on the one hand there must be some causal connection between microevolution and macroevolution. And on the other hand, evolutionary processes operate and drive evolutionary patterns hierarchically. So, it is to a general discussion of hierarchies that we now turn.
References and further reading
Bowler, P. J. 1983. The Eclipse of Darwinism. Johns Hopkins University Press, Baltimore, MD.
Dobzhansky, T. 1937. Genetics and the Origin of Species. Columbia University Press, New York, NY.
Eldredge, N. 1979. Alternative approaches to evolutionary theory. Bulletin of the Carnegie Museum of Natural History 13: 7-19.
Eldredge, N. 1985. Unfinished Synthesis. Oxford University Press, New York, NY.
Eldredge, N. 1989. Macroevolutionary Dynamics: Species, Niches, and Adaptive Peaks. McGraw-Hill, New York, 217 pp.
Eldredge, N. and J. Cracraft. 1980. Phylogenetic patterns and the evolutionary process: method and theory in comparative biology. Columbia University Press, New York, 349 pp.\
Filipchenko, Y. 1927. Variabilität und Variation.
Goldschmidt, R. 1940. The Material Basis of Evolution. Yale University Press, New Haven, CT.
Gould, S. J. 1989. Wonderful Life: The Burgess Shale and the Nature of History. W. W. Norton, New York, NY.
Gould, S. J. 2002. The Structure of Evolutionary Theory. Harvard University Press, Cambridge, MA.
Hendricks, J. R., E. E. Saupe, C. E. Myers, E. J. Hermsen, and W. D. Allmon. 2014. The generification of the fossil record. Paleobiology 40(4): 511-528.
Hennig, W. 1966. Phylogenetic Systematics. University of Illinois Press, Champaign, IL.
Huxley, J. 1942. Evolution, The Modern Synthesis. Allen & Unwin, London.
Jablonski, D. 2017. Approaches to macroevolution: 1. General concepts and origin of variation. Evolutionary Biology 44: 427-450.
Jablonski, D. 2017. Approaches to macroevolution: 2. Sorting of variation, some overarching issues, and general conclusions. Evolutionary Biology 44: 451-475.
Lieberman, B. S., and N. Eldredge. 2014. What is punctuated equilibrium? What is macroevolution? A response to Pennell et al. Trends in Ecology & Evolution 29(4): 185-186.
Lieberman, B. S., W. Miller III, and N. Eldredge. 2007. Paleontological patterns, macroecological dynamics and the evolutionary process. Evolutionary Biology 34(1-2): 28-48.
Mayr, E. 1942. Systematics and the Origin of Species. New York: Columbia University Press.
Myers, C. E., and E. E. Saupe. 2013. A macroevolutionary expansion of the modern synthesis and the importance of extrinsic abiotic factors. Palaeontology 56:1179-1198.
Simpson, G. G. 1944. Tempo and Mode in Evolution. Columbia University Press, New York, NY.
Simpson, G. G. 1953. The Major Features of Evolution. Columbia University Press, New York, NY.
Stanley, S. M. 1979. Macroevolution. W. H. Freeman, San Francisco, CA.
Vrba, E. S. 1980. Evolution, species, and fossils: How does life evolve? South African Journal of Science 76: 61-84.
Vrba, E. S., and N. Eldredge. 1984. Individuals, hierarchies and processes: towards a more complete evolutionary theory. Paleobiology 10: 146-171.
Wiley, E. O. 1981. Phylogenetics: The Theory and Practice of Phylogenetic Systematics. John Wiley & Sons, New York, 439 pp.
Content usage
Usage of text and images created for DEAL: Text on this page was written by Bruce S. Lieberman and Jonathan R. Hendricks. Original written content created by Bruce S. Lieberman and Jonathan R. Hendricks for the Digital Encyclopedia of Ancient Life that appears on this page is licensed under a Creative Commons Attribution-NonCommercial-ShareAlike 4.0 International License. Original images created by Jonathan R. Hendricks are also licensed under Creative Commons Attribution-NonCommercial-ShareAlike 4.0 International License.
Content sourced from other websites: Attribution, source webpage, and licensing information or terms of use are indicated for images sourced from other websites in the figure caption below the relevant image. See original sources for further details. Attribution and source webpage are indicated for embedded videos. See original sources for terms of use. Reproduction of an image or video on this page does not imply endorsement by the author, creator, source website, publisher, and/or copyright holder.