Chapter contents:
Evolution and the Fossil Record
– 1. Natural selection
– 2. Species and species concepts
– 3. Speciation
– 4. Punctuated equilibria and stasis
–– 4.1 Videos about punctuated equilibrium and stasis
– 5. Macroevolution
–– 5.1 Hierarchies ←
–– 5.2 Species selection
–– 5.3 Abiotic vs. biotic causes of macroevolution
–– 5.4 Evolutionary radiations
The genealogical hierarchy, emergence, and the difficulties with extrapolationism
The biological world consists of different kinds of entities and the hierarchical relationships among them are a key quality of biological systems: genes are housed in cells, cells make up organisms, organisms form populations, populations make up species, and species form clades. This is known as the genealogical hierarchy. Almost all of the entities in the hierarchy are things that make more or less faithful copies of themselves, and are in some ways involved with reproduction or "more-making." The exception are clades; even though they don't reproduce, they are formed by the production of new species and for this reason are treated as members of the genealogical hierarchy.

Simplified overview of the genealogical hierarchy of relationships between genes, cells, organisms, populations, species, and clades. Image by Jonathan R. Hendricks.
Niles Eldredge was one of the first scientists to consider the relevance of natural hierarchies to evolution (other prominent scientists who considered this topic were Elisabeth Vrba, Stephen Jay Gould, and David Jablonski). Eldredge published several articles and books on this topic, beginning in the 1980’s, including the 1985 work “Unfinished Synthesis.” As part of his research, Eldredge recognized the connection to—and built on the larger intellectual heritage of—such authors as Theodosius Dobzhansky, who in his 1937 “Genetics and the Origin of Species” remarked that there was a distinction between what he called the physiologies of organisms and the physiologies of populations.
The patterns and processes that connect genes, cells, organisms, and populations encompass microevolution. Within species, these patterns and processes (and within clades these patterns) encompass macroevolution.
Each higher-level entity in the geneological hierarchy is more than just the simple sum of the parts of the entity that exists at the next lower level: species represent more than just a collection of organisms; organisms represent more than just a collection of cells; etc. A term used to describe this phenomenon is “emergence,” which entails a degree of increased complexity and organization. For example, an adult human consists of a billion cells, but one cannot put a billion cells in a blender and produce a human.
Another excellent example comes from the physics of planetary bodies. It is straightforward to mathematically model the position of a single planet revolving around the Sun. Adding in a third planet, however, dramatically increases the complexity, such that it is now no longer easy to model the orbits of the bodies. In fact, it is an incredible mathematical challenge. Indeed, there are many chaotic solutions to the model, such that the behavior is more than just the sum of two separate two-planet systems.
"Weird Orbits - the three body problem" by David Gozzard (YouTube).
Sometimes, however, increasing the number of things does not lead to any emergent complexity. Consider the notorious Professor Chaos, whose stated goal is to spread discord throughout the world.
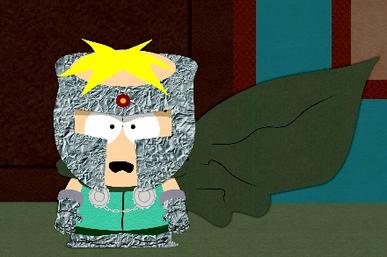
Professor Chaos, the alter ego of character Butters from the television show South Park. Image copyright Comedy Central and Viacom. This image was originally released as part of a collection of promotional media materials and its usage here is interpreted as falling under fair use guidelines.
Imagine that Professor Chaos were cloned, producing an exact duplicate. The world would face the challenge of twice the chaos. There is no reason to assume, however, that Prof. Chaos 1 and Prof. Chaos 2 would—in combination—produce a sort of new, emergent chaos. Nor would the addition of a third Professor Chaos; instead, the amount of chaos wrought would simply triple. Increasing the number of things (here, Professors of Chaos) does not inherently lead to emergence of new properties.
The simple fact that nature is hierarchically structured has major consequences for the manner in which evolution occurs. This is because processes within any one entity do not necessarily extrapolate up to higher levels in the geneological hierarchy.
Junk DNA provides an excellent example of this. Junk DNA comprises regions of the genomes of humans and other organisms that have no apparent effect on cellular function or organismal phenotype. Such non-functional regions of the genome may result from duplication events: a functional gene is copied, then rendered useless by a mutation. There is no way for the non-functional gene to be ejected from the genome, however, so it just "comes along for the ride." These junk genetic regions have neither beneficial nor ill effects on the organism they are contained in, so they are not under organismic natural selection. Indeed, close to 90% of the human genome may be junk DNA (in other words, you may only be a consequence of 10% of your genome). This junk DNA, while comprising a significant portion of the total genome, has no emergent effects on organisms.
Sometimes it is absolutely possible to extrapolate processes at a lower level of organization to explain patterns at a higher level. For instance, the physical behavior of minerals that we can observe in the world directly relates to their molecular structure. Muscovite nicely illustrates this principle, as it is a mineral that cleaves (or, breaks) in sheets in handsample that reflect the underlying chemical structure of the mineral at an atomic level. This can be observed by comparing the two models shown below.
3D photogrammetry model of a handsample of the mineral muscovite. Model by Earth Sciences, University of Newcastle (Sketchfab).
3D model showing the atomic structure of the mineral muscovite. The bonds in the blue-grey planes are especially strong, whereas the bonds connecting these planes are quite weak, explaining why the mineral forms sheets in hand sample. Model by Earth Sciences, University of Newcastle (Sketchfab).
Let's now consider emergence of properties in a biological context using beetles as an example. Beetles are the most diverse group of lifeforms (nearly 30% of all described species are beetles).
The brown rhinoceros beetle, Xylotrupes gideon. Model by "Rigsters" (Sketchfab).
Perhaps beetles are so diverse because individual beetles are unusually fit organisms, making any given beetle species less likely to go extinct, thereby favoring the accumulation of beetle species over time. Perhaps this pattern is not, however, best explained by extrapolation. Instead, maybe the reason there are so many beetles is that beetle species are more likely to speciate when compared to other groups. Organisms, however, do not speciate by themselves; they only do so in the context of other members of their species. Thus, speciation is an emergent property of groups of individual organisms.
Reductionism doesn’t always work in biology and we cannot simply extrapolate from lower to higher levels. This is a result of the fact that distinct levels of organization exist in nature. Sometimes, however, reductionism does work at a macroevolutionary scale. One example was identified by Strotz et al. (2018). These authors demonstrated that organismal physiology directly explained patterns of species extinction and survival in marine mollusk species from the western Atlantic over the last few million years. In contrast to what happens as our focus moves upwards in the biological hierarchy—where we recognize it isn’t always possible to scale up—the patterns and processes at a higher level always control those acting at lower levels. If an organism dies, so do all of the cells within it. If a species goes extinct, so do all of the populations of individual organisms within it.
The ecological hierarchy and its interactions with the genealogical hierarchy
Ecological relationships largely have to do with the biological processing and transfer of energy across levels of organization. Like the genealogical hierarchy, this ecological flow of energy is thought to be organized hierarchically in nature. There is a distinction, however, between phenomena operating in genealogical entities—such as genes, cells, and organisms—and those operating in ecological entities such as communities and regional biotas.
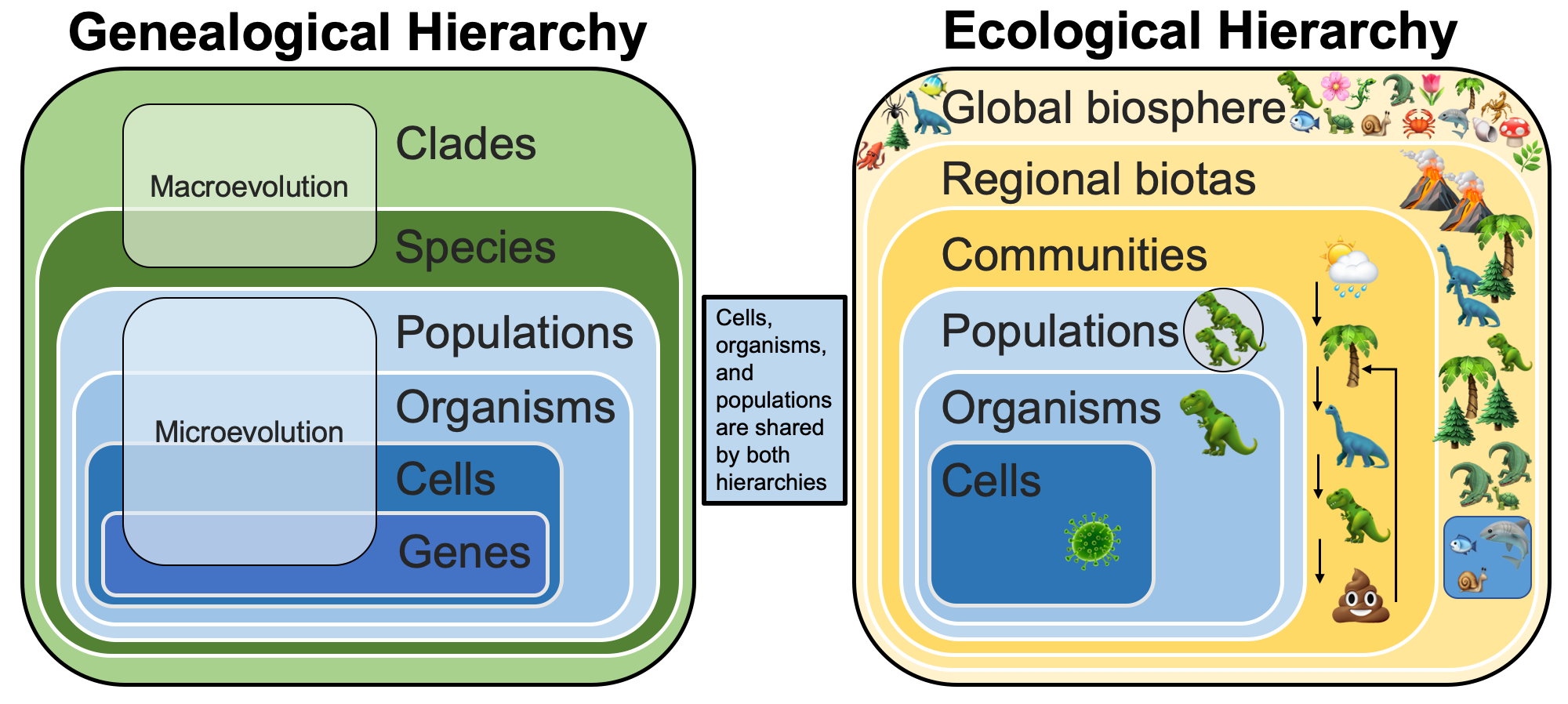
Comparsion of the genealogical and ecological hierarchies. Note that cells, organisms, and populations are shared in both hierarchies.
Communities and regional biotas do not reproduce, nor do they make more of themselves, although the populations and species they contain do. There are some entities, however, that appear in both the genealogical and the ecological hierarchy: cells, organisms, and populations. For instance, animals (organisms) consume food (sometimes each other) and convert it into energy (ecological hierarchy) that is used for both survival and reproduction (genealogical hierarchy).
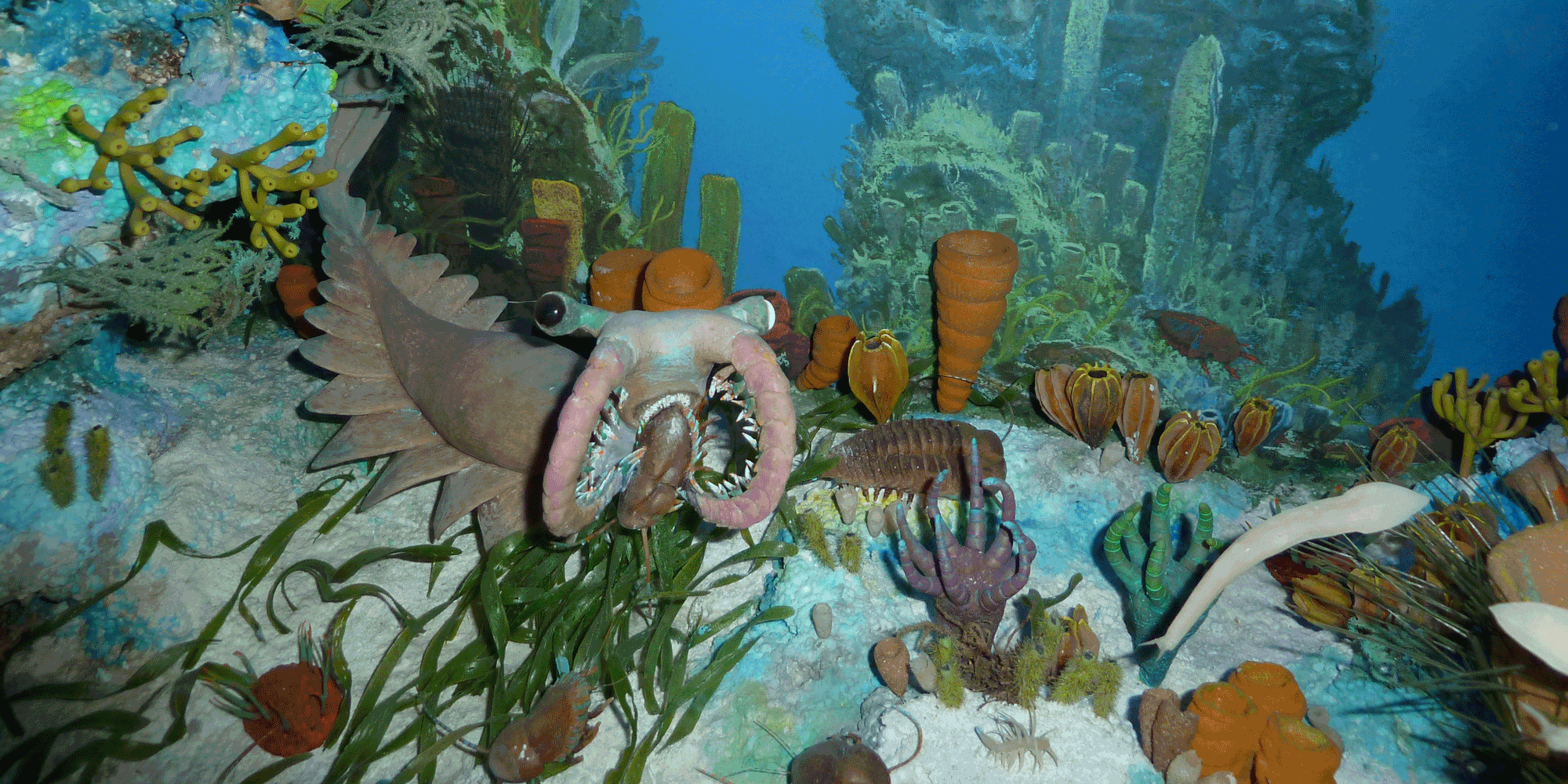
Diorama on display at the Florida Museum of Natural History depicting the Cambrian Burgess Shale ecological community.
The entities in the ecological hierarchy might seem a bit fuzzy relative to those in the genealogical hierarchy (it is easier to define a species than a community). But the same perspectives on causality and the limits of extrapolationism mentioned above apply to entities, patterns, and processes in the ecological hierarchy. Further, the entities that are shared between the two hierarchies—cells, organisms, and populations—provide the direct causal link between ecology and evolution. Entities interact in the ecological arena; what happens there has consequences up or down the ecological hierarchy. These consequences filter across into the genealogical hierarchy via the shared entities, with corresponding upwards and downwards effects. Patterns in lower- or higher-level genealogical entities have to be filtered through ecological changes and—as further remarked upon below—the two hierarchies are not synonymous.
Connections between ecology and macroevolution
Although related, ecology and evolution are separate and this is why patterns in one sphere can be very different from those in another. An excellent example of this comes from the work of McKinney et al. (1998), who showed that the changing patterns of abundance in bryozoan communities over the last 75 million years differed substantially from changes in patterns of taxonomic diversity. Some bryozoan groups that show a dramatic drop in diversity after the end Cretaceous Mass Extinction at the same time show significant increases in abundance. Elisabeth Vrba, in a classic paper (Vrba, 1987), also showed that even though the total number of species of antelopes greatly exceeds the number of species of closely related impalas, the total number of individuals across antelope and impala species was highly congruent. Finally, Niles Eldredge developed his theory of the “Sloshing Bucket” to describe how ecological changes—precipitated by various phenomena, including mass extinctions—have consequences that branch out through the genealogical and ecological hierarchies. Another exciting area of growth in these types of investigations involves work by Alycia Stigall and Erin Saupe, who examine the relationship between genealogical and ecological entities in the fossil record using an analytical approach known as ecological niche modeling (ENM).
Unfortunately, scientists have not always recognized the importance of properly distinguishing between ecological and genealogical entities when formulating evolutionary theory. Indeed, looking back on the history of evolutionary biology in the 20th century, scientists associated with the Neo-Darwinian synthesis (such as Dobzhansky and Simpson) assumed that there was a direct equivalence between ecological and genealogical entities. This is reflected in their presumption that broad taxonomic groups were largely equal to broad adaptive zones, but such a view has been shown to be patently false: entire clades do not interact as a whole with other clades, nor with their environment. Instead, these interactions happen at a much smaller scale (populations within species of those clades). (In a subsequent section we will focus in greater detail on the relative contributions that abiotic and biotic factors have played in shaping the large-scale history of life.)
One of the major developments of the Modern Synthesis of the mid-20th century was the consensus view that all evolution could be viewed as smooth extrapolation from phenomena among the lowest entities of the genealogical hierarchy (genes and organisms) to those at the highest levels (species and clades). We now think, however, that proponents of the Modern Synthesis failed to adequately distinguish between differing phenomena in ecological and genealogical entities and the hierarchies of organization that they entail. This meant that the Modern Synthesis never came to full fruition, nor did it produce a complete picture of how evolution worked (though it was absolutely an advance on theories that had come previously).
References and further reading
Dobzhansky, T. 1937. Genetics and the Origin of Species. Columbia University Press, New York, NY.
Eldredge, N. 1985. Unfinished Synthesis. Oxford University Press, New York, NY.
Eldredge, N. 1989. Macroevolutionary Dynamics, Englewood Cliffs, NJ.
Eldredge, N. 2007 "Hierarchies and the Sloshing Bucket: Toward the Unification of Evolutionary Biology". Evolution: Education and Outreach. 1 (1): 10–15.
Gould, S. J. 2002. The Structure of Evolutionary Theory. Harvard University Press, Cambridge, MA.
Jablonski, D. 2017. Approaches to macroevolution: 2. Sorting of variation, some overarching issues, and general conclusions. Evolutionary Biology 44:451-475.
Lieberman, B. S. 2000. Paleobiogeography: Using Fossils, to Study Global Change, Plate Tectonics, and Evolution. Kluwer Academic/Plenum Publishing, New York, New York.
Lieberman, B. S., W. Miller. III, and N. Eldredge. 2007. Paleontological patterns, macroecological dynamics and the evolutionary process. Evolutionary Biology 34:28-48.
Maguire, K. C., and A. L. Stigall. 2009. Using ecological niche modeling for quantitative biogeographic analysis: a case study of Miocene and Pliocene Equinae in the Great Plains. Paleobiology 35:587-611.
McKinney, F. K., S. Lidgard, J. J. Jr. Sepkoski, and P.D, Taylor. 1998. Decoupled temporal patterns of evolution and ecology in two post-Paleozoic clades. Science 281:807-809.
Myers, C. E., and Saupe, E. E. 2013. A macroevolutionary expansion of the modern synthesis and the importance of extrinsic abiotic factors. Palaeontology 56:1179-1198.
Palazzo, A. F., and T. R. Gregory. 2014. The case for junk DNA. PLoS Genetics 10(5): e1004351.
Pyron, R. A., F. T. Burbrink, and T. J. Guiher. 2008. Claims of potential expansion throughout the U.S. by invasive phython species are contradicted by ecological niche models. PLoS ONE 3(8): e2931.
Saupe, E. E., Q. Huijie, J. R. Hendricks, R. W. Portell, S. J. Hunter, J. Soberón, and B. S. Lieberman. 2015. Niche breadth and geographic range size as determinants of species survival on geological time scales. Global Ecology and Biogeography 24:1159-1169. DOI: 10.1111/geb. 12333 .
Simpson, G. G. 1944. Tempo and Mode in Evolution. Columbia University Press, New York, NY.
Stigall, A. L. 2019. The invasion hierarchy: ecological and evolutionary consequences of invasions in the fossil record. Annual Review of Ecology, Evolution, and Systematics 50:15.1-15.26.
Strotz, L. C., E. E. Saupe, J. Kimmig, and B. S. Lieberman. 2018. Metabolic rates, climate and macroevolution: a case study using Neogene molluscs. Proceedings of the Royal Society B 285(1885).
Valentine, J. W. 1973. Evolutionary Paleoecology of the Marine Biosphere, Prentice-Hall, New York, NY.
Vrba, E. S. 1987. Ecology in relation to speciation rates: some case histories of Miocene-Recent mammal clades. Evolutionary Ecology 1:283-300.
Vrba, E. S., and N. Eldredge. 1984. Individuals, hierarchies and processes: towards a more complete evolutionary theory. Paleobiology 10:146-171.
Zimmer, C. 2015. Is most of our DNA garbage? New York Times, March 5, 2015.
Content usage
Usage of text and images created for DEAL: Text on this page was written by Bruce S. Lieberman and Jonathan R. Hendricks. Original written content created by Bruce S. Lieberman and Jonathan R. Hendricks for the Digital Encyclopedia of Ancient Life that appears on this page is licensed under a Creative Commons Attribution-NonCommercial-ShareAlike 4.0 International License. Original images created by Jonathan R. Hendricks are also licensed under Creative Commons Attribution-NonCommercial-ShareAlike 4.0 International License.
Content sourced from other websites: Attribution, source webpage, and licensing information or terms of use are indicated for images sourced from other websites in the figure caption below the relevant image. See original sources for further details. Attribution and source webpage are indicated for embedded videos. See original sources for terms of use. Reproduction of an image or video on this page does not imply endorsement by the author, creator, source website, publisher, and/or copyright holder.